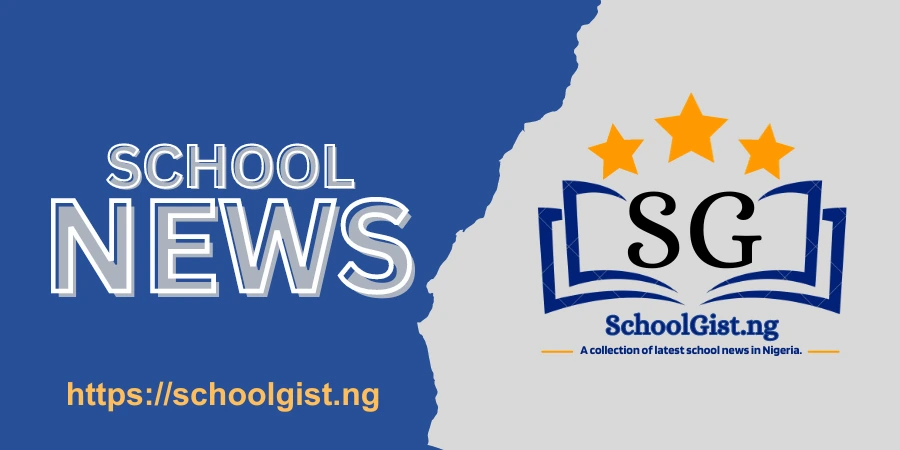
Chemistry lesson note for SS2 Third Term is now available for free. The State and Federal Ministry of Education has recommended unified lesson notes for all Firstary schools in Nigeria, in other words, all private Firstary schools in Nigeria must operate with the same lesson notes based on the scheme of work for Chemistry.
Chemistry lesson note for SS2 Third Term has been provided in detail here on schoolgist.ng
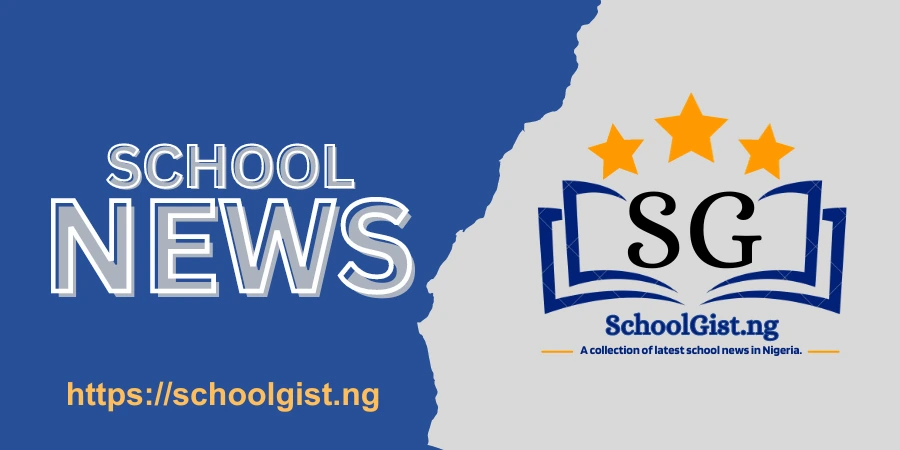
For prospective school owners, teachers, and assistant teachers, Chemistry lesson note is defined as a guideline that defines the contents and structure of Chemistry as a subject offered at SS level. The lesson note for Chemistry for SS stage maps out in clear terms, how the topics and subtopics for a particular subject, group works and practical, discussions and assessment strategies, tests, and homework ought to be structured in order to fit in perfectly, the approved academic activities for the session.
To further emphasize the importance of this document, the curriculum for Chemistry spells out the complete guide on all academic subjects in theory and practical. It is used to ensure that the learning purposes, aims, and objectives of the subject meant for that class are successfully achieved.
Chemistry Lesson note for SS2 carries the same aims and objectives but might be portrayed differently based on how it is written or based on how you structure your lesson note. Check how to write lesson notes as this would help make yours unique.
The SS2 Chemistry lesson note provided here is in line with the current scheme of work hence, would go a long way in not just helping the teachers in carefully breaking down the subject, topics, and subtopics but also, devising more practical ways of achieving the aim and objective of the subject.
The sudden increase in the search for SS2 Chemistry lesson note for Third Term is expected because every term, tutors are in need of a robust lesson note that carries all topics in the curriculum as this would go a long way in preparing students for the West African Secondary Examination.
This post is quite a lengthy one as it provides in full detail, the Chemistry-approved lesson note for all topics and sub-topics in Chemistry as a subject offered in SS2.
Please note that Chemistry lesson note for SS2 provided here for Third Term is approved by the Ministry of Education based on the scheme of work.
I made it free for tutors, parents, guardians, and students who want to read ahead of what is being taught in class.
SS2 Chemistry Lesson Note (Third Term) 2024
SCHEME OF WORK FOR SS2 CHEMISTRY 3RD TERM
WEEKS TOPICS
- SOURCES AND TYPES OF WATER
- STRUCTURE OF WATER & AND VOLUME RELATIONSHIPSHARDNESS OF WATER
- SOLUBILITY
- MASS AND VOLUME RELATIONSHIPS
- ACID –BASE TITRATION
- INTRODUCTION TO ORGANIC CHEMISTRY
- ALKANES
- ALKENES
- ALKYNES & BENZENE
- ALKANOLS
WEEK 1
INTRODUCTION
SOURCES AND TYPES OF WATER
In the Earth’s biosphere, water is essential to all forms of life. Interestingly, it is also the biospheres most abundant compound and a unique substance from the chemical point of view.
About 75% of the Earth’s surface consists of water. Water is present as either (a) Solid – ice, snow etc., (b) Liquid – water in the lakes, rivers and oceans etc., (c) Water vapour – as clouds or moisture in the air.
The Human body contains as much as 70% water and all animals and vegetable matter contain water; plants contain up to 50-70% of water.
Of the total estimated global water supply of 1.4 x 109km3, fresh water sources amount to only 2.7% of the total. Most fresh water is not readily available, being under the ground, or in the form of glaciers and frozen lakes. The fraction of water available for human civilization is estimated at less than 0.003% of the total global water availability. Aquatic marine life in oceans, seas and other saline inland water bodies, enjoy 97.3% of the total global supply of water supply.
Hard Water
- Hard Water. This is saturated with calcium, iron, magnesium, and many other inorganic minerals. All water in lakes, rivers, on the ground, in deep wells, is classified as hard water. (Many city systems take water from rivers or lakes, or reservoirs supplied with mountain water; they erroneously call their supplies “soft water” but it is soft only in comparison with water which is harder.)
Boiled Water
- Boiled Water. Boiling helps remove some of the germs, but concentrates the inorganic minerals. Other germs are carried into a fertile element for rapid and lusty propagation of germs and viruses already in the body.
Raw Water
- Raw Water. This has not been boiled. Raw water may be hard (as calcium hardened water) or soft as rain water. It contains millions of germs and viruses. In every densely inhabited drop. Some of these viruses and bacteria may adversely affect the thyroid gland, the liver and other vital body organs.
Rain Water
- Rain Water. This has been condensed from the clouds. The first drop is distilled water. But when it falls as rain, it picks up germs, dust, smoke, minerals, strontium 90, lead and many other atmospheric chemicals. By the time rain water reaches the earth it is so saturated with dust and pollutants it may be yellowish in color. Water is supposed to act as an atmosphere purifier. If we had no air pollution, we would have far less pollution in our drinking water.
Snow Water
- Snow Water. This is frozen rain. Freezing does not eliminate any germs. All snowflakes have hardened mineral deposits. Melt the cleanest snow and you will find it saturated with dirt, inorganic minerals, germs and viruses.
Filtered Water
- Filtered Water. This water has passed through a fine strainer, called a filter. Some calcium and other solid substances are kept in the filter; there is no filter made which can prevent germs from passing through its fine meshes. Each pore of the finest filter is large enough for a million viruses to seep through in a few moments. A home filter usually only picks up suspended solids and is effective for the time, maybe only for hours, until it is filled up. Then it is ineffective even for removing suspended solids, and at the same time becomes a breeding ground for bacteria.
Soft Water
- Soft Water. This water is soft in comparison with water which is harder. It may contain many trace minerals and chemicals, viruses and bacteria. It is not to be confused with “softened water.” Soft water may be classified as water which is harder than distilled water.
Reverse Osmosis
- Reverse Osmosis. This is a system of water purification which allows pre-filtered water to be forced through a semi-permeable membrane to separate impurities from our drinking water. However, this membrane allows only certain molecules to pass through providing the water pressure is exactly constant. The matter of water pressure is a problem still to be solved. Furthermore, the membrane also allows some iron and nitrate molecules to pass through. Another problem to be solved.
But it now seems promising that we can look forward to tremendous strides in this system of water purification. Gulf Oil, Culligan and Eastman Kodak are sponsoring vast research in this area. High purity water between 90 and 97 percent of the dissolved mineral (and organic solutions), and over 99 percent of the suspended and colloidal particles are rejected by, the special membrane which has been developed for this process by these companies in their research. The product water, from this new research, is ultra-clear, low in dissolved solids, practically free from hardness components and essentially sterile as produced. This makes a close second to distilled water.
De-ionized Water
- De-ionized Water. A process of exchanging “hard” ions for “soft.” The total ions are still present. The end result is the same. But the water has the appearance of being distilled. (Nature recognizes transformation but not extinction!) Since water leaving the sodium-cation exchanger has little hardness, it contains sodium salts.
Distilled Water
- Distilled Water. This is water that has first been turned into steam so that all of its impurities are left behind. Then through condensation, it is turned back into pure water. It is the only pure water. The only water free from all contamination. Distilled water may well be considered the only pure water on earth.
Water Pollution
Based on source of pollution, the following types of water pollution are recognised
- Water pollution due to city wastes
- Water pollution due to agriculture
- Industrial water pollution
- Water pollution due to natural reasons
Based on severity of pollution, the water pollution could be classified as
- Dangerous / serious water pollution
- Mild and rectifiable water pollution
- Negligible water pollution
Quite frequently, a water pollution could find a place in two or more groups. For e.g., an agricultural water pollution may be serious and industrial water pollution may be mild or sea water may be polluted by agricultural chemical and underground water may be polluted by city wastes.
Although all the above groupings are meaningful, it is more convenient if the classification based on utility and location of water is considered for detailed discussion. In this classification, drinking water pollution can be caused by its source and during its path of movement. Thus, the ground water pollution and river water pollution may ultimately lead to drinking water pollution when ground water or river is polluted. River water and underground water can lead to many other complications other than drinking water pollution alone. It is to be observed that sea water is significantly polluted, although no pollutant is directly added to sea (except accidental ship leakage or sinking of ship etc). This is because, all polluted rivers lead to pollutants to sea and due to continuous accumulation, the concentration of pollutants in sea water gets increased as the time passes.
Important Features of Water Pollution
- Water pollution can be point source or non – point source. That is, it can be caused near the source of pollution or where source of pollution is sometimes far away from source.
- Nature also contributes to water pollution besides human activities.
- The concentration of pollutants is generally on reducing trend, as the water moves to longer distances / depths and as the time passes.
- Not all pollutants in water are dangerous. And not all dangerous pollutants are really harmful. Few pollutants are not dangerous immediately. But they may cause ill effects after long time.
- The sensitivity to pollutants in drinking water is more connected to physiology of human / animal body than concentration of pollutant within safe range.
Major Water Pollutants
Current water pollution issues can be divided into the following categories:
- Sewage and other oxygen-demanding wastes,
- Infectious or disease causing agents,
- Plant nutrients,
- Synthetic organic chemicals,
- Inorganic minerals and chemical compounds,
- Suspended solids or sediments,
- Radioactive substances,
- Thermal discharges,
- Oil,
- Detergents.
Sewage and other oxygen-demanding waste
Oxygen-consuming wastes comprise of raw sewage from municipalities and other wastes from paper mills, tanneries, food-processing plants and agriculture; these cause a proliferation of aerobic decomposers who consume dissolved oxygen (DO) in water more rapidly than it can be replaced by the atmosphere. Organic matter undergoes oxidation in rivers under the action of micro-organisms.
Biochemical oxygen demand (BOD) is a measure of demand for oxygen utilized by micro-organism, during oxidation of organic matter. It is defined as the amount of oxygen in milligram per liter or in ppm used by micro-organisms to degrade the organic matter. A high BOD value indicates more polluted water.
The dissolved oxygen in rivers and other water bodies is usually sufficient to decompose only small or treated discharge of such waste. The products formed during aerobic decomposition e.g. CO2, SO42-, NH4+ or NO3- ions do not cause much pollution.
If, however, the amount of sewage and other wastes are heavy, then the dissolved oxygen (DO) is insufficient and oxidation of organic matter is incomplete. Incomplete oxidation results in the formation of amines, ethane, H2S, etc., which produce wormy smell. It also depletes the dissolved oxygen (DO) in water to levels, which cannot sustain aquatic life. If the level of dissolved O2 falls below 5 ppm (parts per million), fish begin to die.
When we treat our sewage with chlorine to kill bacteria, we also kill marine ecosystems with the chlorine itself, or with carcinogenic chlorinated hydrocarbon by products.
Infectious or disease causing agents
These are the various pathogenic micro-organisms which may enter the water along with sewage or other wastes. These microbes, mainly bacteria and viruses, can cause various diseases such as cholera, typhoid, dysentery, gastroenteritis, polio, hepatitis etc. in the water supply drawn from these sources. The bacterium found in sewage (E-coli) has been known to affect shellfish and salmon populations in USA and causes severe food poisoning and gastroenteritis.
Plant nutrients
The presence of plant nutrients in lakes and slow-moving waters supports high population of aquatic plants that on decay, deplete dissolved oxygen (DO) making the survival of aquatic life problematic. Besides this, these dead plants also produce disagreeable odor.
The enrichment of water by nutrients is known as ‘eutrophication’. Lakes and slow-moving waters age through eutrophication and over periods of several millennia, they get converted into swamps and marshes.
Synthetic organic compounds
Pesticides, detergents, chemical dyes and other industrial chemicals and their waste constitute synthetic organic compounds. When present in water, these chemicals can act as toxic poisons for plants, animals and humans. Such chemicals enter the hydrosphere either by usage, by accidental or intentional disposal of wastes from manufacturing units and by losses during their transport. In the USA at the turn of the century, lobster die-off occurred on Long Island. It may be linked to the runoff of the pesticide malathion, used to eradicate the West Nile virus-carrying mosquitoes, since crustaceans are closely related to insects.
Some laundry detergents and fertilizers such as nitrogen, phosphorus and other nutrients cause an overgrowth of algae, bacteria, and aquatic plants. This also leads to ‘eutrophication’ and eventually the lake can no longer support life.
Acid rain, water pollution
Acid rain
The oxides of nitrogen and sulphur are corrosive and poisonous. When in excess in the atmosphere, these gases react with water (e.g., rain water) to form acids, and thus result into acid rain.
Acid rain has the following harmful effects:
- It damages the nutrition level of leaves of the plants.
- Acid rain promotes corrosion.
- It damages the structures made of marble, cement and / or lime viz., historical monuments.
- Acid rain harms the aquatic life viz., it kills fish etc.
- Acid rain leads to the loss of soil fertility.
Chemistry of acid rain
Normally while rain travels through the air, it dissolves floating chemicals and washes down particles that are suspended in air. At the start of its journey raindrops are neutral (pH = 7). In clean air, rain picks up materials that occur naturally such as dust, pollen, some CO2 and other chemicals produced by lightening or volcanic activities. These substances make rain slightly acidic (pH = 6), which is not dangerous. However, when rain falls through polluted air, it comes across chemicals such as gaseous oxides of sulphur (SOx), oxides of nitrogen (NOx), mists of acids such as hydrochloric and phosphoric acid, released from automobile exhausts industrial plants, electric power plants etc.
These substances dissolve in falling rain making it more acidic than normal with pH range between 5.6 -3.5. In some case, it’s pH gets lowered to the extent of 2. This leads to acid rain. The term acid rain is used here to describe all types of precipitation, namely, rain, snow, fog and dew more acidic than normal.
In the natural processes of volcanic eruptions, forest fires and bacterial decomposition of organic oxides of sulphur and nitrogen, production and reductions of gases naturally tend to an equilibrium. Power plants, smelting plants, industrial plants, burning of coal and automobile exhausts, release additional sulphur dioxide, nitrogen oxides and acidic soot, causing pollution. Sulphur dioxide and nitrogen dioxide interact with water vapours in presence of sunlight to form sulphuric acid and nitric acid mist.
The formed sulphuric acid and nitric acid remain as vapour at high temperatures. These begin to condense as the temperature falls and mix with rain or snow, on the way down to the Earth and make rain sufficiently acidic.
Harmful effects of acid rain
SOx, NOx mixed with water as acid rain causes plant, animal and material damage. Some of the significant ill effects of acid rain are:
Damage to animals
Acid rain chemically strips waterways of necessary nutrients and lowers the pH to levels where plants and animals cannot live. Most of the aquatic animals cannot survive when the pH is less than 4. Some species of fish, such as salmon, die even when the pH is less than 5.5. Certain species of algae and zooplankton are eliminated at pH less than 6. A reduction in the zooplankton and bottom fauna ultimately affects the food availability for the fish population. The problem is most severe downwind of industrial areas where fishing and tourism are major sources of income such as in Norway and Sweden.
Damage to plants
Acidic water is dangerous to plants. Sulphuric and nitric acid rain washes nutrients out of the soil, damages the bark and leaves of trees and harms the fine root hairs of many plants which are needed to absorb water. Leaf pigments are decolorized because acid affects green pigment (chlorophyll) of plants. Agricultural productivity is also decreased. Several non-woody plants, such as barley, cotton and fruit trees like apple, pear, etc., are severely affected by acid rain. Since the acid concentration increases near the base of clouds by density, high altitude trees and vegetation may be exposed to pH levels as low as 3. Unique areas such as the Black Forest in Germany and sugar maples in Vermont (USA) are particularly threatened.
Material damage
Metallic surfaces exposed to acid rain are easily corroded. Textile fabrics, paper and leather products lose their material strength or disintegrate by acid rain.
Building materials such as limestone, marble, dolomite, mortar and slate are weakened on reaction with acid rains because of the formation of soluble compounds.
Thus, acid rain is dangerous for historical monuments.
Water is so valuable to the entire system of the human body that it is wise to use only the Best. Use pure steam distilled water for health and well being.
REVISION EXERCISES
- List three properties of water.
- List five uses of water
- Mention two types of water.
WEEK 2
STRUCTURE OF WATER
Structure of water molecule in the gas phase
A molecule of water consists of two hydrogen atoms joined to an oxygen atom by covalent bonds.
The oxygen atom has six electrons (1s2 2s2 2p4) in its outermost shell. The ‘s’ and ‘p’ orbitals of the valence shell are sp3 hybridized to form four sp3 hybrid orbitals oriented tetrahedrally around the oxygen atom. Two of the hybrid oribtals are singly occupied, while the lone pairs of electrons occupy the other two. Each singly occupied sp3 orbital overlaps with the half-filled 1s orbital of ‘H’ atom. Thus, oxygen is bonded to the two hydrogen atoms by two O-H covalent bonds, and there are two lone-pairs of electrons on the oxygen atom. Due to the presence of two lone-pairs of electrons on the O atom, the H-O-H bond angle is 104.5°, which is slightly less than the tetrahedral angle of 109°28′. Therefore, the structure of water molecule is an angular or bent structure.
Polarity of water molecule
Oxygen is more electronegative than hydrogen, being only second to fluorine in its electronegativity. Its high electronegativity causes the oxygen atom to pull the shared pairs of electrons more towards itself. As a result, the O-H bond acquires polarity. Since the two O-H bonds in water are inclined at an angle, hence the net dipole moment of water molecule is not zero. The actual dipole moment of water molecule is 1.84 debye, (denoted as D) is the unit of dipole moments.
Structure of water molecule in the liquid state
In liquid water, water molecules are held together by intermolecular hydrogen bonds. Each oxygen atom can form two hydrogen bonds utilizing both the lone pairs on it. Experimental studies suggest that liquid water consists of aggregates of varying number of water molecules held together by hydrogen bonds and ‘free’ water molecules in a dynamic equilibrium. The aggregates continually forming, collapsing and reforming. Thus, in liquid water, the equilibrium
Hydrogen bonding has very profound effect on the properties of water.
Structure of water in solid state (ice)
Depending on the conditions for freezing of water, the solid form of water i.e., ice, can exist in different crystalline forms. In ‘normal’ hexagonal ice, four other oxygen atoms tetrahedrally surround each oxygen atom. One hydrogen atom lies in between each pair of oxygen atoms. Thus, each and every hydrogen atom is covalently bonded to a oxygen atom and linked to another oxygen atom by a hydrogen bond. This arrangement leads to a packing with large open spaces and results in a lower density of ice than that of liquid water.
When ice melts some of the hydrogen bonds are broken and the water molecules become more closely packed. It results in an increase in the density of water above its melting points (273 K). Density of water attains a maximum value of 1 g/mL at 277 K (4°C); above 277 K, the density decreases due to the normal temperature effects.
Fig: 11.4 – Structure of normal hexagonal ice
There is one hydrogen along each oxygen – oxygen axis closer to one of the two oxygen atoms.
Solvent property of water
The polarity of water
Water has a simple molecular structure. It is composed of one oxygen atom and two hydrogen atoms. Each hydrogen atom is covalently bonded to the oxygen via a shared pair of electrons. Oxygen also has two unshared pairs of electrons. Thus there are 4 pairs of electrons surrounding the oxygen atom, two pairs involved in covalent bonds with hydrogen, and two unshared pairs on the opposite side of the oxygen atom. Oxygen is an “electronegative” or electron “loving” atom compared with hydrogen.
Water is a “polar” molecule, meaning that there is an uneven distribution of electron density. Water has a partial negative charge () near the oxygen atom due the unshared pairs of electrons, and partial positive charges () near the hydrogen atoms.
An electrostatic attraction between the partial positive charge near the hydrogen atoms and the partial negative charge near the oxygen results in the formation of a hydrogen bond as shown in the illustration.
The ability of ions and other molecules to dissolve in water is due to polarity. For example, in the illustration below sodium chloride is shown in its crystalline form and dissolved in water.
Many other unique properties of water are due to the hydrogen bonds. For example, ice floats because hydrogen bonds hold water molecules further apart in a solid than in a liquid, where there is one less hydrogen bond per molecule. The unique physical properties, including a high heat of vaporization, strong surface tension, high specific heat, and nearly universal solvent properties of water are also due to hydrogen bonding. The hydrophobic effect, or the exclusion of compounds containing carbon and hydrogen (nonpolar compounds) is another unique property of water caused by the hydrogen bonds. The hydrophobic effect is particularly important in the formation of cell membranes. The best description is to say that water “squeezes” nonpolar molecules together.
- at pH 7.0, a solution is neutral
- at lower pH (1-6), a solution is acidic
- at higher pH (8-14), a solution is basic
TESTING FOR WATER PURITY
oIt is possible to do some simple tests to detect certain ions in water, though these tests described, suitable for doing in schools and colleges, are not effective in detecting very low concentrations.
oNever-the-less you can do simple precipitation tests e.g.
- using barium chloride to detect sulfate ions,
- and using silver nitrate to detect halide ions – chloride, bromide and iodide.
REVISION EXERCISES
- What causes hardness of water?
- Mention two types of hardness of water.
- Mention two ways of removing temporary hardness of water.
- What causes permanent hardness of water?
- List two advantages of hard water.
- List two disadvantages of hard water.
- Describe two ways of testing for water in the laboratory.
- Give an equation to show what happens when slaked lime is added to temporary hard water.
WEEK 3
SOLUBILITY
Definition of Terms
Solute
This is a substance that dissolves in a solvent to form a solution. It can be solid, liquid or gas. Examples include common salt, sugar, copper (II) tetraoxosulphate (VI) etc.
Solvent
This is a substance that dissolves a solute to form a solution. It can be liquid or gas. Examples are water, ethanol, benzene etc.
Solution
This is a mixture of solute and solvent, i.e,
Solute + Solvent = Solution
It can be homogeneous with a uniform composition, e.g, an unsaturated solution of a sodium chloride, or heterogeneous with non-uniform composition like an unsaturated solution of the same salt.
Solution is defined as a homogeneous mixture of two or more chemical substances. The state of matter of a solution may be solid, liquid or gaseous.
For example, common salt in water (liquid solution), air (gaseous solution), alloys (solid solution), etc.
The components of a solution may be classified as:
- Solute
- Solvent
Difference between solute and solvent
Thus, an intimate mixture of solute and solvent is called a solution.
Aqueous solutions and non-aqueous solutions
Aqueous solutions
Solutions that contain water as the solvent are called aqueous solutions.
For example, sugar in water, carbon dioxide in water, etc.
Non-aqueous solutions
Solutions that contain a solvent other than water are called non-aqueous solutions. Ether, benzene, petrol, carbon tetrachloride etc., are some common solvents.
For example, sulphur in carbon disulphide, naphthalene in benzene, etc.
Concentrated solutions and dilute solutions
Between two solutions, the solute quantity may be relatively more or less. The solution that has a greater proportion of solute is said to be more concentrated than the the other that has a lesser proportion. If the proportion of solute is less, the solution is said to be dilute.
Saturated Solution
A saturated solution is one that holds as much solute as it can dissolve in the presence of undissolved solute particles at a given temperature. In a saturated solution, the dissolved and undissolved solutes are in equilibrium at a given temperature.
Unsaturated Solution
An unsaturated solution is one that can still dissolve more solutes at a given temperature until it becomes saturated.
For instance, the solubility limit of sodium chloride is 36g dm^-3, which means that as long as the mass of sodium chloride in 1dm^3 of its solution is below 36g, the solution will remain unsaturated. However, immediately the mass is 36g and above, the solution will be considered to be saturated because any mass in excess of 36g will be precipitated out of solution as undissolved particles. This implies that if the mass of sodium chloride in the solution is 37g; 1g which is the excess will be precipitated out as undissolved particles.
Supersaturated Solution
A solution which contains more of the solute than it can normally hold at a given temperature is said to be supersaturated. The supersaturated solution is unstable at a given temperature and the solute particles can crystallize out of the solution at the slightest touch or addition of a dry crystal of the solute. At times, dust particles can cause the crystallization of the solute particles. Also, merely scratching the inside of the glass vessel holding the solution with a glass rod or spatula can cause the solute particles to crystallize out. This is known as seeding, and is employed in crystallization as a separation technique. Solution and solubility
Solubility of a solute
Solubility is defined as the number of grams of a solute that dissolves in 100g of a solvent to form a saturated solution at a given temperature and pressure.
Solubility is the maximum weight of a solute that can be dissolved in 100g of a solvent at a given temperature and pressure.
Factors affecting the solubility of a solid solute in water
Temperature
Increase in temperature increases the solubility.
For example, it is easier to dissolve sugar in hot milk than in cold milk.
Size of solute particles
Smaller the size of the particles greater is the solubility.
For example, it is easier to dissolve powdered sugar than granules of sugar.
Mechanical stirring
Mechanical stirring increases solubility.
For example, sugar dissolves faster on stirring with a spoon.
Factors affecting the solubility of a gas in water
Temperature
Increase in temperature, decreases the solubility of a solute. On heating, the gases dissolved in milk escape making the milk bubble and boil over.
Pressure
An increase in pressure increases the solubility of a gas.
For example, aerated water bottles contain carbon dioxide gas under pressure.
Influence of Pressure on the Solubility of Gases
Pressure directly influences the solubility of gases. Higher the pressure, higher is the solubility: lower the pressure, lower is the solubility. For instance, the solubility of carbon dioxide in water, under normal atmospheric pressure is very low. But when subjected to higher pressure it dissolves to a great extent, as in the case of aerated waters.
Remember
Speed of Dissolving of a Solid in a Liquid
From our day-to-day experience, we come to know that a solid can dissolve faster if:
- a) The particles are smaller.
- b) The mixture is stirred or agitated.
- c) The solvent is at a higher temperature.
So the fastest way to dissolve a solid in a liquid is to grind the solid into very tiny particles, stir the mixture, and use a hot solvent. Dissolving Bournvita in a hot cup of milk is a good example!
Determination of the solubility of a solid in water at a given temperature
If you want to determine the solubility of a solid, say potassium nitrate in water, at any given temperature, this is how you should proceed:
- a) Take a clean dry evaporating dish, and find its weight, as accurately as possible. Let the weight of the empty evaporating dish be ‘a’ gm.
- b) Take a clean beaker and a glass rod. Pour about 20-25 ml of distilled water into the beaker. Warm the water to 2oCto 3oc above the temperature, at which you are determining the solubility (i.e., if you are determining the solubility at 40oC, warm it upto 42oC to 43oC). Keep on adding small quantities of potassium nitrate with constant stirring with the glass rod, till no more of it dissolves. Now stop warming it, but keep on stirring, till the temperature comes down to the required level-say 40oC. Carefully transfer about 10-15 ml of this solution into the previously weighed evaporating dish. Care has to be taken to see that no crystals are transferred.
Find the weight of the evaporating dish and the saturated solution.
Let this weight = ‘b’ gm.
- c) Place the dish with the saturated solution, on a sand bath and carefully evaporate the water. Cool the dish to room temperature and find the weight of the dish and the solute. To make sure that all the water has been evaporated, heat the dish again for 3 to 5 minutes, then cool and weigh. Repeat the heating, cooling and weighing several times, till two consecutive weights are the same.
Let this weight be ‘c’ g.
- d) Observations
Weight of clean dry evaporating dish = ‘a’ g
Weight of dish + saturated solution = ‘b’ g
Wight of dish + solute = ‘c’ g
- e) Calculations
Weight of solute alone = (c – a) g
Weight of solvent alone = (b – c) g
Solubility of solute in solvent at that temperature
Solubility curve:
The solubility of a given solute in water, as done above, can be determined at many different temperatures, say 0oC, 10oC, 20oC,…..100oC. The result so obtained can be plotted on a graph by taking temperatures along the X-axis, and solubility along the Y-axis. By joining the points so obtained, you get a curve, called the solubility curve. The solubility curves of six solutes in water are given in the graph.
Observe the graph carefully and notice the following, regarding the solubility of these six solutes in water.
- a) Sodium nitrate has the highest solubility at 0oC.
- b) Potassium chlorate has the lowest solubility at 0oC.
- c) Potassium nitrate has the highest solubility at 100oC.
- d) The solubility of potassium nitrate is low at 0oC but increases drastically with increase in temperature.
- e) The solubility of sodium chloride is very slightly affected by the increase in temperature; the increase in solubility is only 4.1 grams from 0oC to 100oC.
DEDUCTIONS of solubility curve:
- a) It gives a general idea about the ability of a solute to dissolve in water, at different temperatures.
- b) The solubility of the solute at any temperature, between the given lowest and highest temperatures, can be more or less accurately read.
- c) The mass of crystals deposited, when a saturated solution so cooled from a higher to a lower temperature, can be calculated.
For e.g.,
As per the given graph, the solubility of potassium nitrate at 60oC= 110 g and at 50oC = 91 g.
Hence, by cooling a saturated solution of potassium nitrate containing 100 g of water from 60oC to 50oC, 19 g of the solute (110 g – 91 g) is deposited in the form of crystals.
Applications of Solubility Curves
- a) They are used in pharmaceutical companies to determine the amounts of drugs that must be dissolved in a particular quantity of solvent to give a prescribed drug mixture.
- b) They are used by chemists and other research workers to determine the most suitable solvents that can be used to extract essential oils from their natural sources at various temperatures. Example of an essential oil is the eucalyptus oil.
- c) It is applied in fractional crystallization.
Calculations on Solubility
Mathematically, solubility can be expressed as:
Solubility (mol dm^3) = No. of moles/Volume (dm3)…………(i)
= (No. of moles/Volume (cm3)) x 1000………(ii)
Equations (i) and (ii) are used when the volume is given in dm^3 and cm^3 respectively.
Also,
Solubility (g dm^-3) = Reacting mass/Volume (dm3)…………(iii)
= (Reacting mass/Volume (cm3)) x 1000………(iv)
but,
No. of moles = Reacting mass/Molar mass…………(v)
Substituting equation (v) into (i) and (ii), then;
Solubility (mol dm-3)
= (Reacting mass/Molar mass) x 1/Volume (dm3)……(vi)
= (Reacting mass/Molar mass) x 1000/Volume (cm3)……(vii)
Equations (vi) and (vii) are used when the molar mass with the reacting mass is given.
.
Examples
Question 1
In an experiment to determine the solubility of a given salt Z, the following data were obtained:
Mass of empty dish = 14.32g
Mass of dish + saturated solution of salt Z = 35.70g
Mass of dish + salt Z = 18.60g
Temperature of solution = 30°C
Molar Mass of salt Z = 100
Density of solution Z = 1.00g cm-3
Determine the solubility of salt Z in (i) g dm-3 (ii) mol dm-3
Answer
Mass of saturated solution
= Mass of dish + saturated solution of salt Z – Mass of empty dish
= 35.70g – 14.32g
= 21.38g
Mass of salt Z = Mass of dish + salt Z – Mass of empty dish
= 18.60g – 14.32g
= 4.28g
Molar Mass of salt Z = 100
Amount in moles of salt Z = Mass of salt Z/Molar mass of salt Z
= 4.28/100
= 0.0428 mole
Density of solution Z = 1.00g cm-3
Volume of solution = Mass of solution/Density of solution
= 21.38g /1g cm-3
= 21.38 cm3
Therefore, solubility of salt Z in:
- i) g dm-3 = (Mass/Volume (cm3)) x 1000
= (4.28/21.38) x 1000
= 200.19g dm-3
- ii) mol dm-3 = (Moles/Volume (cm3)) x 1000
= (0.0428/21.38) x 1000
= 2.00mol dm-3
Question 2
16.55g of lead (II) trioxonitrate (V) was dissolved in 100g of distilled water at 20°C, calculate the solubility of the solute in mol per dm3. [Pb = 207, N = 14, O = 16]
Answer
Mass of Pb(NO3)2 = 16.55g
Molar mass of Pb(NO3)2 = (1xPb) + 2[(1xN) + (3xO)]
= (1 x 207) + 2[(1 x 14) + (3 x 16)]
= 207 + 2(14 + 48)
= 207 + 124
= 331g mol-1
Volume of water = 100cm3 (density of water = 1g cm-3)
Solubility (mol dm-3) = (Reacting mass/Molar mass) x 1000/Volume (cm3)
= (16.55/331) x (1000/100)
= 0.05mol dm-3
Question 3
Potassium trioxochlorate (V) has a solubility of 1.8 mol per dm3 at 35°C. On cooling this solution to a temperature of 20°C, the solubility was found to be 0.3 mol per dm3. What mass of KClO3 was crystallized out? [K = 39, Cl = 35.5, O = 16]
Answer
Molar mass of KClO3 = (1xK) + (1xCl) + (3xO)
= (1 x 39) + (1 x 35.5) + (3 x 16)
= 39 + 35.5 + 48
= 122.5g mol-1
Solubility of salt at 35°C = 1.8mol dm-3
Solubility of salt at 20°C = 0.3mol dm-3
Amount in moles of KClO3 crystallized out on cooling from 35°C – 25°C = 1.8 – 0.3
= 1.5 moles
Therefore, mass of salt crystallized out on cooling = Amount in moles x Molar mass
= 1.5 X 122.5
= 183.75g
Question 4
The solubility of a salt of molar mass 101g at 35°C is 0.35 mol per dm3. If 3.50g of the salt is dissolved completely in 250cm-3 of water in beaker, what will the resulting solution be – unsaturated, saturated or supersaturated?
Answer
Solubility of salt at 35°C = 0.35mol dm-3
Molar mass of salt = 101g
Mass of salt in solution = 3.50g
Volume of water = 250cm3
Solubility of salt in saturated solution = (Reacting mass/Molar mass) x 1000/Volume (cm^3)
= (3.5/101) x (1000/250)
= 0.138mol dm^-3
Since the calculated solubility of the salt solution in the beaker at 35°C, is less than the standard solubility of the salt at the same temperature, it means that the salt has not reached its solubility limit at that given temperature. Hence, the resulting solution will be unsaturated.
Question 5
The solubility of Na3AsO4.12H2O is 38.9g per 100g H2O. What is the percentage of Na3AsO4 in the saturated solution? [As = 75, Na = 23, O = 16, H = 1]
Answer
Molar mass of Na3AsO4.12H2O = (3xNa) + (1xAs) + (4xO) + 12[(2xH) + (1xO)]
= (3 x 23) + (1 x 75) + (4 x 16) + 12[(2 x 1) + (1 x 16)]
= 69 + 75 + 64 + 12(2 + 16)
= 208 + 216
= 424g mol-1
From the above, molar mass of Na3AsO4 = 208g mol-1
Mass of Na3AsO4.12H2O in 100g of water = 38.9g
Volume of water = 100cm3 (density of water – 1g cm-3)
Therefore, solubility of Na3AsO4.12H2O (g dm-3)
= (Mass/Volume (cm3 )) x 1000
= (38.9/100) x 1000
= 389g dm-3
but,
424g of Na3AsO4.12H2O contains 208g of Na3AsO4
Therefore,
389g of Na3AsO4.12H2O will contain x of Na3AsO4
x = (389 x 208)/424
= 190.83g of Na3AsO4
Percentage of Na3AsO4 in the saturated solution
= (Mass of Na3AsO4 in solution/Mass of Na3AsO4.12H2O in solution) x 100
= (190.83/389) x 100
= 49.1%
Question 6
80g of a salt, XCl2, was placed in
40cm3 of water to give a saturated solution at 25°C. If the solubility of the salt is 8 mol dm-3 at that temperature, what is the mass of the salt that will be left undissolved at the given temperature? [X = 24, Cl = 35.5]
Answer
Molar mass of XCl2 = (1xX) + (2xCl)
= (1 x 24) + (2 x 35.5)
= 24 + 71
= 95g mol-1
At 25°C,
Solubility of XCl2 = 8.0mol dm-3
i.e, 1 dm3 of solution contains 8 x 95 = 760g
If, 1000cm3 of water = 760g of XCl2
Then, 40cm3 of water = x g of XCl2
x = 760 x 40/1000
= 30.4g of XCl2
From the above, based on the solubility of the salt, 40cm3 will only dissolve 30.4g
Therefore, the mass of the salt that will be left undissolved is 80 – 30.4 = 49.6g
Question 7
The solubility of potassium trioxochlorate (V) is 1500g per 1000g water at 80°C and 600g per 1000g water at 45°C. Calculate the mass of KClO3 that will crystallize out of solution if 200g of the saturated solution at 80°C is cooled to 45°C.
Answer
Masses at 80°C,
1000g of water + 1500g of KClO3 = 2500g of saturated solution
Masses at 45°C,
1000g of water + 600g of KClO3 = 1600g of saturated solution
Mass of salt deposited on cooling from 80°C to 45°C
= 2500 – 1600 = 900g
If 2500g of saturated solution deposit 900g of solute on cooling
Then, 200g of the saturated solution will deposit x g of solute
x = 900 x 200/2500
= 72g of KClO3.
Do These
Question 1
If 24.4g of lead (II) trioxonitrate (V) were dissolved in 42g of distilled water at 30°C; calculate the solubility of the solute in g per dm3
Question 2
Calculate the solubility in mol per dm3 of 50g of CuSO4 dissolved in 100g of water at 120°C. [Cu = 64, S = 32, O = 16]
Question 3
If 10.5g of lead (II) trioxonitrate (V) in 20cm3 of distilled water at 18°C, what is the solubility of the solute in mol per dm^3?
Question 4
117g of NaCl was dissolved in 1dm3 of distilled water at 25°C. Determine the solubility in mol per dm3 of sodium chloride at that temperature. [Na = 23, Cl = 35.5]
Question 5
- a) What do you understand by terms saturated solution and solubility?
- b) When 50cm3 of a saturated solution of sugar of molar mass 345g at 50°C was evaporated to dryness, 34.5g of dry solid was obtained. Calculate the solubility of sugar ay 50°C in a) g per dm3 b) mol per dm3
Question 6
3.06g of a sample of potassium trioxochlorate (V), KClO3, was required to make a saturated solution with 10cm3 of water at 25°C. What is the solubility of the salt at that temperature?
REVISION EXERCISES
- Differentiate between true solution and false solution.
- Define solubility.
- What is meant by the term saturated solution ?
- What is meant by supersaturated solution?
- What is the solubility of a solute in a given solvent?
- 25.0g of a solute are required to saturate 80.0g of water at 25oC.Calculate its solubility.
WEEK 4
MASS AND VOLUME RELATIONSHIP
Mole concept is arguably the broadest topic in chemistry, as it cuts across every other branch of chemistry, as far as reactions are concerned. It is the foundation of calculations in chemistry.
Chemical reactions, expressed as equations, are ways of confirming the Law of Conservation of Mass, which states that matter is neither created nor destroyed, but is transformed from one form to another. This is also what was paraphrased in one of the postulations of Dalton’s Atomic Theory, which is, atoms are neither created nor destroyed (during a chemical reaction), but are changed from one form to another.
The above implies that if two substances A and B combine to give another substance C, i.e,
A + B —> C
then, the total masses of A and B will equal the mass of C. In other words, all of A and B will be converted to C, assuming there is no loss. Meaning that if we know the masses of A and B, we can easily calculate what we should be expecting as C.
For instance, in the reaction between sodium and chlorine to form sodium chloride;
2Na + Cl2 —> 2NaCl
Before reacting (reactants):
Na = 2*1 = 2 atoms
Cl = 1*2 = 2 atoms
After reacting (products):
Na = 2*1 = 2 atoms
Cl = 2*1 = 2 atoms
we can see that 2 atoms of Na, combined with 2 atoms of Cl to form 2 moles of NaCl, made up of 2 atoms of Na and 2 atoms of Cl each; and if we carry out atom counts, we will observe that no atom was created (added)and none was destroyed (lost).
From the above example, it means that whenever Na and Cl combine to form NaCl, they MUST do so in the ratio of 2:1:2 That is to say, 2 moles of Na molecule will always combine with 1 mole of Cl molecule to form 2 moles of NaCl molecule. This is known as the stoichiometry of the reaction, (i.e, the relationship between the amounts of the reactants and the products, in terms of the mole ratio and mass ratio of the species involved).
Definition of Terms
Mole
Since the substances that react contain different amounts of elementary particles (atoms, molecules, ions, electrons etc), which directly or indirectly contribute to their masses; it is always difficult to measure the exact amount of a particular substance that may be required to react with another. Thus, the need to take their masses and volumes (if gaseous), to “the same base”. This “base” is known as the mole.
Hence, a mole can be defined as the amount of elementary entities or particles (atoms, molecules, ions, electrons etc) present in a substance, which is exactly as those present in 12 grammes of carbon-12.
Molar Mass
This is the mass of 1 mole of a substance expressed in grammes. Its is expressed in grammes per mole (g/mol).
Molar Volume
This is the volume occupied by 1 mole of a substance, usually gases, at standard temperature and pressure (s.t.p). It is also known as the molar gas volume (G.M.V), and has a constant value of 22.4dm^3 or 22400cm^3.
Avogadro Number
This is a constant value of 6.023 x 10^23, which represents the amount of elementary entities present in 1 mole of a substance. It can be likened to a dozen – 12, a score – 20, or a gross – 144.
Important Formulae Used in Mole Concept
1) Mole (n) = Reacting Mass (m)
Molar Mass (M)
i.e, n = m/M
This implies that,
Reacting Mass = Mole x Molar Mass
Molar Mass = Reacting Mass/Mole
2) Mole (n) = Reacting Volume (v)
Molar Volume (V)
i.e, n = v/V
This implies that,
Reacting Volume = Mole x Molar Volume
Molar Volume = Reacting Vol/Mole
3) Mole (n) = Number of Particles
6.023 x 10^23
Relationship Between Mole & Mass (m), Volume (v) and Avogadro Number (L)
The relationships between the four quantities stated above can be expressed as shown below:
Molar Mass — Mole — Molar Volume
|
Avogadro Number
i.e, 1 mole = 6.023 x 10^23 = 22.4dm^3 = molar mass
1mole of CO2 = 44g of CO2 = 22.4dm^3 of CO2 = 6.023 x 10^23 molecules of CO2
1mole of O2 = 32g of O2 = 22.4dm^3 of O2 = 6.023 x 10^23 molecules of O2 = 2 x 6.023 x 10^23 atoms
1mole of Na = 23g of Na = 6.023 x 10^23 molecules of Na = 6.023 x 10^23 atoms of Na.
Example:
2Na(s) + Cl2(g) —> 2NaCl(s)
You will observe that we have introduced the state symbols (s – solid, g – gas), which enables us to know the physical states at which the reacting species and products are at room temperature. Chemical calculation based on mass volume relationship
Example:
Calculate the volume of carbon dioxide formed at STP in ‘ml’ by the complete thermal decomposition of 3.125 g of pure calcium carbonate (Relative atomic mass of Ca=40, C=12, O=16)
Solution:
As per the equation,
40 + 12 + (16 x 3) 1 mole
100g 22.4 litres
100 g 24400 ml
Volume of carbon dioxide formed from 100 g of CaCO3 = 22400 ml
Volume of carbon dioxide from 3.125 g of CaCO3 = ?
CaCO3 : CO2
100 g : 22400 ml
3.125 g : x
Volume of carbon dioxide formed = 700 ml.
Example:
Calculate the volume of ammonia formed at STP in ‘ml’ by treating 2.675 g of ammonium chloride with excess of calcium hydroxide (Relative Atomic Mass of N=14, O=16, H=1, Cl=35.5, Ca=40).
Solution:
As per the equation,
2 (14 + 4 + 35.5) 2 vols.(of NH3)
107 2 x 22.4 litres
107 g 44.8 litres or 44800 ml
NH4Cl : NH3
107 g : 44800 ml
2.675 g : x
Volume of ammonia formed at STP = 1120 ml.
Example: 31Calculate the volume of sulphur dioxide formed at STP in ‘ml’, by treating 4.8 g of copper with excess of hot concentrated sulphuric acid. (Relative atomic mass of Cu=64, H=1, S=32, O=16).
Solution:
As per the equation,
64 g 1 volume (of SO2)
64 g 22400 ml
Volume of SO2 formed using 64 g of copper = 22400 ml
Volume of SO2 formed using 4.8 g of copper = ?
Cu : SO2
64 g : 22400 ml
4.8 g : x
Volume of sulphur dioxide formed at STP = 1680 ml.
Example:
Calculate the volume of water gas obtained by passing steam over 96 kg of white hot coke (Relative atomic mass of C=12, H=1, O=16).
Solution:
As per the equation,
12 g 1 vol. + 1 vol.
12 g (22.4 + 22.4) litres
12 g 44.8 litres
Volume of water gas formed from 12 g of coke = 44.8 litres
Volume of water gas formed from 96 kg of coke =?
C : Water gas
12 g : 44.8 litres
96000 g : x litres
Volume of water gas formed = 358400 litres.
olar volume is the volume occupied by one mole of any gas at a definite pressure and temperature. It is denoted by Vm. Molar volume of the substance depends on temperature and pressure. The unit of molar volume is litre per mol or millilitre per mol.
As per Avogadro’s law, equal volumes of all gases contain equal number of molecules, at a constant temperature and pressure. Therefore, equal number of molecules of any gas, must occupy the same volume, at constant temperature and pressure.
Standard Molar Volume
Standard molar volume of a gas is the volume occupied by 1 mole of any gas at 273 K and 1 atm pressure (STP). It is equal to 22.4 litres of 22,400 ml. It is the same for all gases.
Remember
S.T.P. = Standard Temperature and Pressure
Standard Temperature = 0oC or 273 K
Standard Pressure = 1 atm or 760 mm of mercury
Calculation of Molar Volume
Example of oxygen
Mass of 1 litre of oxygen at STP = 1.429 g
Mass of 1 mol of oxygen = 32 g
Volume : Mass
1 litre : 1. 429 g
x : 32 g
The following table gives the relation between the Gram Molecular Weight (GMW), Number of moles, Molar Volume and the Number of particles for gases at STP.
Relationship between Various Parameters of Gases, at STP
Gas Molecular Formula GMW
(in g) No.Of Moles Molar Volume
dm3 or l No.of moles in 1 mole
Hydrogen H2 2 1 22.4 6.023x 1023
Oxygen O2 32 1 22.4 6.023x 1023
Nitrogen N2 28 1 22.4 6.023x 1023
Chlorine Cl2 71 1 22.4 6.023x 1023
Carbon dioxide CO2 44 1 22.4 6.023x 1023
Nitrogen dioxide NO2 46 1 22.4 6.023x 1023
Ammonia NH3 17 1 22.4 6.023x 1023
Methane CH4 16 1 22.4 6.023x 1023
Sulphur dioxide SO2 64 1 22.4 6.023x 1023
Problems Based on Molar Volume
Example:
Calculate the volume occupied by 3.4 g of ammonia at STP. (N=14, H=1)
Solution
Gram molecular mass of ammonia (NH3) = (1 x 14) + (3 + 1) = 14 + 3 = 17 g
Mass of 1 mol of ammonia = 17g
Molar volume = 22.4 litres
Volume of 3.4 g of ammonia at STP = ?
Mass : Volume
17 g : 22.4 litre
3.4 g : x
Volume occupied by 3.4 g of ammonia at STP =4.48 litres
Example:
56 ml of carbon dioxide has a mass at 0.11 g at STP. What is the molar mass of the carbon dioxide?
Solution:
Mass of 56 ml of carbon dioxide at STP = 0.11 g
Mass of 22400 ml of carbon dioxide = ?
Mass : Volume
0.11 g : 56 ml
x g : 22400 ml
Mass of 22400 ml of carbon dioxide at STP = 44 g
Molar Mass of carbon dioxide = 44 g/mole.
Example:
One gram of pure sulphur dioxide has a volume of 350 ml at STP. What is the Relative Molecular Mass of sulphur dioxide?
Solution:
Volume of sulphur dioxide gas = 350 ml at STP
Mass of sulphur dioxide gas = 1 g
Mass of one mole of sulphur dioxide = x g/mole
Volume of 1 mole of sulphur dioxide = 22400 ml at STP
Mass : Volume
1 : 350 ml
x : 22400 ml
Mass of 1 mole of SO2 = 64 g/mole
\ Relative molecular mass of sulphur dioxide = 64.
Example:
100 ml of carbon monoxide, has a mass of 0.125 g at STP. Calculate the mass of 1 mole of carbon monoxide.
Solution:
Volume of carbon monoxide = 100 ml
Mass of carbon monoxide = 0.125 g
Molar volume = 22400 ml
Mass of 1 mole of carbon monoxide =?
Volume : Mass
100 ml : 0.125 g
22400 ml : x g
Mass of one mole of carbon monoxide = 28 g/mole
\ Relative molecular mass of carbon monoxide = 28.
Deduction of relationship between molecular mass and vapour density
Relative Molecular Mass
Relative molecular mass is the ratio of the mass of one molecule of a substance to the mass th of a carbon atom, or 1 amu.
Vapour Density
Vapour density is the ratio of the mass of a volume of a gas, to the mass of an equal volume of hydrogen, measured under the same conditions of temperature and pressure.
According to Avogadro’s law, equal volumes of all gases contain equal number of molecules.
Thus, let the number of molecules in one volume = n,
Cancelling ‘n’ which is common, we get
However, since hydrogen is diatomic.
When we compare the formulae of vapour density with relative molecular molecular mass, they can be represented as
We can therefore substitute the above equation, and arrive at the below formula
Now on cross multiplication, we have
2 x vapour density = Relative Molecular Mass of a gas
or,
Relative Molecular Mass = 2 x Vapour density
Mole concept
Since it is not possible to calculate the weight of particles individually, a collection of such particles called mole is taken for all practical purposes. Avogadro discovered that under standard conditions of temperature and pressure, (1atm and 273K) a sample of gas occupies a volume of 22.4 L.
It was discovered that the number of atoms present in 12g of carbon is 6.023 x 1023 atoms. This is referred to as Avogadro number.
A mole of a gas is the amount of a substance containing 6.023 x 1023 particles.
Avogadro’s number is the number of atoms present in C12 isotope = 12g of C.
One mole of any gas at STP will have a volume of 22.4 L.
Mole in term of mass, volume number and ions
In chemistry, the term mole represents a pile or mass of atoms, molecules, ions or electrons. Just as common man measures quantity in terms of kilograms or dozens, a chemical scientist deals with a ‘mole’ of atoms, molecules, ions or electrons.
Mole is defined as the amount of a substance, which contains the same number of chemical units (atoms, molecules, ions or electrons) as there are atoms in exactly 12 grams of pure carbon-12. A mole represents a collection of 6.023 x 1023 ( Avogadro’s number) chemical units. Thus a mole represents the quantity of material, which contains one Avogadro’s number of chemical units of any substance. The unit of mole is denoted as ‘mol’.
For example
One mole of hydrogen atoms = 6.023 x 1023 atoms of hydrogen
One mole of hydrogen molecules = 6.023 x 1023 molecule of hydrogen
One mole of electrons = 6.023 x 1023 electrons
One mole of sodium ions (Na+) = 6.023 x 1023 Na+ ions
It can be thus concluded that,
One mole of atoms = 6.023 x 1023 atoms = Gram atomic mass of the element.
One mole of molecules = 6.023 x 1023 molecules = Gram molecular mass.
Molar Volume
The volume occupied by one mole of any substance is called its molar volume. It is denoted by Vm. Molar volume of the substance depends on temperature and pressure. One mole of all gaseous substances at 273 K and 1 atm pressure occupies a volume equal to 22.4 litre or 22,400 mL. The unit of molar volume is litre per mol or millilitre per mol.
The various relationships of mole can be summarized as follows:
Calculation based on mole concept
Problems
- Calculate the average atomic weight of silicon having 92.2% of Si-28 isotope of relative mass 27.98 amu, 4.7% of Si-29 isotope of relative mass 28.98 amu and 3.1% of Si-30 isotope of relative mass 29.97 amu.
Solution
Data given: 92.20% of silicon of mass 27.98 amu
4.7% of silicon of mass 28.98 amu
3.1 % of silicon of mass 29.97 amu
Average atomic weight
= 25.80 + 1.36 + 0.93
= 28.09 amu.
Average atomic weight of silicon is 28.09 amu.11. One million silver atoms weigh 1.79 x 1016 g. Calculate the gram atomic mass of silver.
Solution
Number of silver atoms = 1 million = 1 x 106
Mass of one million Ag atoms = 1.79 x 1016 g
= 107.8 g
Gram atomic mass of silver is equal to the mass of 6.023 x 1023 atoms of silver. So, the gram atomic mass of silver is 107.8 g
- Chlorophyll, the green colouring matter of plants responsible for photosynthesis, contains 2.68% of magnesium by weight. Calculate the number of Mg atoms in 2.00 g of chlorophyll.
Solution
100 g of chlorophyll contains 2.68 g of Mg
1 mole of Mg = 24 g of Mg = 6.023 x 1023 atom of Mg
24 g of Mg contains, 6.023 x 1023 atom of Mg
=1.34 x 1021 Mg atoms
- A sample of gaseous substance weighing 0.5 g occupies a volume of 1.12 litre under NTP conditions. Calculate the molar mass of the substance.
Solution
1 mole of any gaseous substance at NTP occupies 22.4 L.
1.12 L of gaseous substance = 0.5 g
The molar mass of the substance therefore is 10 g/mol.
Firstly, let’s calculate the molar masses:
Molar mass of Na = 1* Relative Atomic Mass of Na
= 1* 23 = 23
Thus, 1mole of Na weighs 23 g/mol.
Molar mass of Cl2 = 2* Relative Atomic Mass of Cl
= 2 * 35.5 = 71
Hence, 1mole of Cl2 molecule weighs 71 g/mol.
Similarly, Molar mass of NaCl = (1* Relative Atomic Mass of Na) + (1* Relative Atomic Mass of Cl)
= (1* 23) + (1 * 35.5)
= 23 + 35.5 = 58.5
Therefore, 1mole of NaCl molecule weighs 58.5 g/mol.
Now, let’s fly:
2Na(s) + Cl2(g) —> 2NaCl(s)
Mole ratio 2 : 1 : 2
Molar mass 23 71 58.5
Molar vol – 22.4dm^3 –
Rxting mass 223 171 2*58.5
46g 71g 117g
(*Rxting Mass = Reacting Mass)
From the above, it means that if we were to prepare sodium chloride in the lab, we would need 46g of sodium metal and 71g of chlorine gas, which would give us 117g of sodium chloride.
Also, remember that chlorine is a gas at room temperature and therefore, possesses molar volume. This implies that 46g of sodium metal can also react with 22.4dm^3 of chlorine gas to form 117g of sodium chloride at s.t.p.
The question now is what if we do not have up to this amounts of reagents in the lab, does it mean we will not be able to prepare sodium chloride? Of course, we will. Let’s say, we have:
(i) 35g of sodium, and
(ii) 50g of chlorine in the lab, how much sodium chloride can we prepare from each?
According to the equation:
2Na(s) + Cl2(g) —> 2NaCl(s)
(i) If 46g of Na produce 117g of NaCl
Then, 35g of Na will produce xg of NaCl
Solve for x by cross multiplying:
46 * x = 117 * 35
46x = 4095
x = 4095/46
= 89.02g of NaCl
(ii) If 71g of Cl produce 117g of NaCl
Then, 50g of Cl will produce xg of NaCl
71 * x = 117 * 50
71x = 5850
x = 5850/71
= 82.39g of NaCl
We can see from the above that we can achieve whatever we desire in the lab, irrespective of the amount of reagents available. All we need is the stoichiometry of the reaction, and every other thing will fall into place.
There is something l want us to note in our calculations above:
In (i), we ignored Cl, while in (ii), we ignored Na. I like calling species like these, ‘distractions’, because though, they are part of the equation, the question did not provide any information about them in each case. In other words, the questions were just silent about them.
Also, we made use of simple proportions in our calculations, by using the relationship between the two species involved in each question, i.e, Na and NaCl in question (i), and Cl and NaCl in question (ii)
Beginners Steps to Take When Solving Problems Involving Mole Concept
1) Write out the balanced chemical equation.
2) Get the stoichiometry or mole ratio of the reaction.
3) Calculate the molar masses of the substances involved.
4) Calculate the molar volumes of the gaseous substances involved (if any).
5) Calculate the reacting masses/volumes of the substances as the case may be.
6) Identity the ‘distractions’ in the equation and ignore them, based on the question(s).
7) Apply simple proportion in solving the problem using the reacting masses/volumes/no. of particles.
Examples
Question 1
Calculate the number of moles present in 8g of oxygen gas (O = 16)
Answer
Oxygen gas, O2 is a diatomic molecule, i.e, it is made up of two atoms.
Reacting mass = 8g
Molar mass = 2 x Relative Atomic Mass of O
= 2 x 16 = 32g/mol
but,
No of moles = Reacting mass
Molar mass
= 8/32 = 0.25mole
Question 2
Calculate the number of
(i) atoms (ii) molecules
present in 35g of nitrogen gas [N = 14, Avogadro No = 6.02 x 10^23/mol]
Answer
Nitrogen gas, N2 is also a diatomic molecule. Before calculating the number of particles in a substance, first calculate the molar mass.
Molar mass of N2 = 2 x 14
= 28g/mol
Reacting mass = 35g
No of moles = Reacting mass
Molar mass
= 35/28 = 1.25moles
remember,
1 mole of any substance = 6.02 x 10^23 entities or particles
(i) N2 contains 2 moles of atoms (diatomic)
Thus, 1mole of N2 = 2 x 6.02 x 10^23 atoms
= 1.204 x 10^24 atoms
Therefore,
If 1mole of N2 = 1.204 x 10^24 atoms
Then, 1.25 moles of N2 = x atoms
x = 1.25 x 1.204 x 10^24
= 1.505 x 10^24 atoms
(ii)N2 is 1 mole of a molecule of nitrogen, and 1 mole of N2 = 6.02 x 10^23 molecules. Therefore,
If 1mole of N2 = 6.02 x 10^23 molecules
Then, 1.25 moles of N2 = x molecules
x = 1.25 x 6.02 x 10^23
= 7.525 x 10^23 molecules
Question 3
What volume in cm^3 will be occupied by 45g of ozone at s.t.p? [O = 16, G.M.V = 22.4dm^3]
Answer
Ozone, O3 is a triatomic molecule, i.e, it is made up of three atoms.
Reacting mass = 45g
Molar mass = 3 x Relative Atomic Mass of O
= 3 x 16 = 48g/mol
but,
No of moles = Reacting mass
Molar mass
= 45/48 = 0.938mole
1 mole = 22.4dm^3 or 22400cm^3
(Since the answer is expected in cm^3, then we will use 22400cm^3)
So,
If 1mole of O3 gas = 22400cm^3
Then, 0.938mole of O3 = x cm^3
x = 0.938 x 22400
= 21011.2cm^3
Therefore, 0.938mole of ozone will occupy 21011.2cm^3.
Question 4
C3H8(g) + 5O2(g) —> 4H2O(g) + 3CO2(g)
From the equation above, calculate the volume of oxygen at s.t.p. required to burn 50cm^3 of propane. [C = 12, H = 1, O = 16, Molar gas volume at s.t.p. = 22.4dm^3]
Answer
From the equation and the accompanying question, all the species are in the gaseous phase, and as such, we will work with their volumes. In other words, we do not need to waste our time calculating their molar masses, so we make use of the relationship between mole and volume.
C3H8(g) + 5O2(g) —> 4H2O(g) + 3CO2(g)
Mole ratio 1 : 5 : 4 : 3
Molar vol 22.4 22.4 22.4 22.4
Rxting vol (1×22400) (5×22400) (4×22400) (3×22400)
= 22400 112000 89600 67200
Therefore, from the stoichiometry,
22400cm^3 of propane = 112000cm^3 of oxygen
Then, 50cm^3 of propane = x cm^3 of oxygen
Solve for x by cross multiplying
22400 * x = 50 * 112000
22400x = 5600000
x = 5600000/22400
= 250cm^3
Therefore, 250cm^3 of oxygen will be required to burn 50cm^3 of propane.
Question 5
2Na(s) + 2H2O(l) —> 2NaOH(aq) + H2(g)
From the equation above, calculate the mass of sodium hydroxide produced by 2.3g of sodium [H = 1, O = 16, Na = 23].
Answer
2Na(s) + 2H2O(l) —> 2NaOH(aq) + H2(g)
Mole ratio 2 : 2 : 2 : 1
Molar mass 23 18 40 2
Rxting mass 46 32 80 2
From the stoichiometry,
46g of Na produce 80g of NaOH
Then, 2.3g of Na will produce xg of NaOH
Solving for x gives,
46 * x = 2.3 * 80
46x = 184
x = 184/46
= 4g
Therefore, 4g of sodium hydroxide will be produced by 2.3g of sodium.
Question 6
16.8g of sodium hydrogentrioxocarbonate (IV) is completely decomposed by heat. Calculate the volume of carbon (IV) oxide given off at s.t.p. [Na = 23, C = 12, O = 16, H = 1, Molar volume of a gas at s.t.p = 22.4dm^3]
Answer
Equation of reaction:
2NaHCO3(s) —> Na2CO3(s) + H2O(l) + CO2(g)
(Note that when a hydrogentrioxocarbonate (IV) salt, [HCO3]- is heated, it decomposes to give a trioxocarbonate (IV) salt, [CO3–], water and carbon (IV) oxide)
Molar masses,
NaHCO3 = (1Na) + (1H) + (1C) + (3O)
= (123) + (11) + (112) + (316)
= 23 + 1 + 12 + 48
= 84g/mol
CO2 = (1C) + (2O)
= (112) + (216)
= 12 + 32
= 44g/mol
(We will focus only on the two compounds, whose molar masses are calculated above. The others [Na2CO3 and H2O] are considered as ‘distractions’, because nothing was mentioned about them in the question)
Mole ratio 2 : 1 : 1 : 1
Molar mass 84 – – 44
Molar vol – – – 22.4
Rxting mass 168 – – 44
Rxting vol – – – 22.4
(Since there is a relationship between mass and volume using the mole, based on the question, we can directly compare the reacting mass of NaHCO3 to the reacting volume of CO2)
Hence, from the stoichiometry;
168g of NaHCO3 = 22.4dm^3 of CO2
16.8g of NaHCO3 = x dm^3 of CO2
168 * x = 16.8 * 22.4
168x = 376.32
x = 376.32/168
= 2.24dm^3
Therefore, 2.24dm^3 of CO2 is liberated at s.t.p. when 16.8g of NaHCO3 decomposes on heating.
Application of Mole Concept
The following are some of the areas where mole concept is applied:
1) Gay-Lussac’s Law
2) Avogadro’s Law
3) Water of Crystallization
4) Law of Conservation of Mass
5) Calculation of Masses from Chemical Equations
6) Redox Reactions
7) Acid-Base Reactions
8) Organic Chemistry
Do These
Question 1
How many moles of limestone, CaCO3 will be required to produce 5.6g of quicklime, CaO?
CaCO3(s) —> CaO(s) + CO2(g)
[Ca = 40, C = 12, O = 16]
- 0.20 B. 0.10 C. 1.12 D. 0.56
Question 2
CaCO3(s) + 2HCl(aq) —> CaCl2(aq) + H2O(l) + CO2(g)
What volume of gas is evolved at s.t.p. if 2g of calcium trioxocarbonate (IV) is added to a solution of hydrochloric acid? [Ca = 40, C = 12, O = 16, Cl = 35.5, H = 1, Molar volume of a gas at s.t.p. = 22.4dm^3]
- 112cm^3 B. 224cm^3 C. 448cm^3 D. 2240cm^3
Question 3
If a solution contains 4.9g of tetraoxosulphate (VI) acid, calculate the amount of copper (II) oxide that will react with it. [Cu = 64, O = 16, S = 32, H = 1]
- 0.8g B. 4.0g C. 40.0g D.
80g
Chemical calculation based on mass volume relationship
Example:
Calculate the volume of carbon dioxide formed at STP in ‘ml’ by the complete thermal decomposition of 3.125 g of pure calcium carbonate (Relative atomic mass of Ca=40, C=12, O=16)
Solution:
As per the equation,
40 + 12 + (16 x 3) 1 mole
100g 22.4 litres
100 g 24400 ml
Volume of carbon dioxide formed from 100 g of CaCO3 = 22400 ml
Volume of carbon dioxide from 3.125 g of CaCO3 = ?
CaCO3 : CO2
100 g : 22400 ml
3.125 g : x
Volume of carbon dioxide formed = 700 ml.
Example:
Calculate the volume of ammonia formed at STP in ‘ml’ by treating 2.675 g of ammonium chloride with excess of calcium hydroxide (Relative Atomic Mass of N=14, O=16, H=1, Cl=35.5, Ca=40).
Solution:
As per the equation,
2 (14 + 4 + 35.5) 2 vols.(of NH3)
107 2 x 22.4 litres
107 g 44.8 litres or 44800 ml
NH4Cl : NH3
107 g : 44800 ml
2.675 g : x
Volume of ammonia formed at STP = 1120 ml.
Example: 31Calculate the volume of sulphur dioxide formed at STP in ‘ml’, by treating 4.8 g of copper with excess of hot concentrated sulphuric acid. (Relative atomic mass of Cu=64, H=1, S=32, O=16).
Solution:
As per the equation,
64 g 1 volume (of SO2)
64 g 22400 ml
Volume of SO2 formed using 64 g of copper = 22400 ml
Volume of SO2 formed using 4.8 g of copper = ?
Cu : SO2
64 g : 22400 ml
4.8 g : x
Volume of sulphur dioxide formed at STP = 1680 ml.
Example:
Calculate the volume of water gas obtained by passing steam over 96 kg of white hot coke (Relative atomic mass of C=12, H=1, O=16).
Solution:
As per the equation,
12 g 1 vol. + 1 vol.
12 g (22.4 + 22.4) litres
12 g 44.8 litres
Volume of water gas formed from 12 g of coke = 44.8 litres
Volume of water gas formed from 96 kg of coke =?
C : Water gas
12 g : 44.8 litres
96000 g : x litres
Volume of water gas formed = 358400 litres.
MOLAR VOLUME.
Molar volume is the volume occupied by one mole of any gas at a definite pressure and temperature. It is denoted by Vm. Molar volume of the substance depends on temperature and pressure. The unit of molar volume is litre per mol or millilitre per mol.
As per Avogadro’s law, equal volumes of all gases contain equal number of molecules, at a constant temperature and pressure. Therefore, equal number of molecules of any gas, must occupy the same volume, at constant temperature and pressure.
STANDARD MOLAR VOLUME
Standard molar volume of a gas is the volume occupied by 1 mole of any gas at 273 K and 1 atm pressure (STP). It is equal to 22.4 litres of 22,400 ml. It is the same for all gases.
Remember
S.T.P. = Standard Temperature and Pressure
Standard Temperature = 0oC or 273 K
Standard Pressure = 1 atm or 760 mm of mercury
Calculation of Molar Volume
Example of oxygen
Mass of 1 litre of oxygen at STP = 1.429 g
Mass of 1 mol of oxygen = 32 g
Volume : Mass
1 litre : 1. 429 g
x : 32 g
The following table gives the relation between the Gram Molecular Weight (GMW), Number of moles, Molar Volume and the Number of particles for gases at STP.
Relationship between Various Parameters of Gases, at STP
Gas Molecular Formula GMW
(in g) No.Of Moles Molar Volume
dm3 or l No.of moles in 1 mole
Hydrogen H2 2 1 22.4 6.023x 1023
Oxygen O2 32 1 22.4 6.023x 1023
Nitrogen N2 28 1 22.4 6.023x 1023
Chlorine Cl2 71 1 22.4 6.023x 1023
Carbon dioxide CO2 44 1 22.4 6.023x 1023
Nitrogen dioxide NO2 46 1 22.4 6.023x 1023
Ammonia NH3 17 1 22.4 6.023x 1023
Methane CH4 16 1 22.4 6.023x 1023
Sulphur dioxide SO2 64 1 22.4 6.023x 1023
Problems Based on Molar Volume
Example:
Calculate the volume occupied by 3.4 g of ammonia at STP. (N=14, H=1)
Solution
Gram molecular mass of ammonia (NH3) = (1 x 14) + (3 + 1) = 14 + 3 = 17 g
Mass of 1 mol of ammonia = 17g
Molar volume = 22.4 litres
Volume of 3.4 g of ammonia at STP = ?
Mass : Volume
17 g : 22.4 litre
3.4 g : x
Volume occupied by 3.4 g of ammonia at STP =4.48 litres
Example:
56 ml of carbon dioxide has a mass at 0.11 g at STP. What is the molar mass of the carbon dioxide?
Solution:
Mass of 56 ml of carbon dioxide at STP = 0.11 g
Mass of 22400 ml of carbon dioxide = ?
Mass : Volume
0.11 g : 56 ml
x g : 22400 ml
Mass of 22400 ml of carbon dioxide at STP = 44 g
Molar Mass of carbon dioxide = 44 g/mole.
Example:
One gram of pure sulphur dioxide has a volume of 350 ml at STP. What is the Relative Molecular Mass of sulphur dioxide?
Solution:
Volume of sulphur dioxide gas = 350 ml at STP
Mass of sulphur dioxide gas = 1 g
Mass of one mole of sulphur dioxide = x g/mole
Volume of 1 mole of sulphur dioxide = 22400 ml at STP
Mass : Volume
1 : 350 ml
x : 22400 ml
Mass of 1 mole of SO2 = 64 g/mole
\ Relative molecular mass of sulphur dioxide = 64.
Example:
100 ml of carbon monoxide, has a mass of 0.125 g at STP. Calculate the mass of 1 mole of carbon monoxide.
Solution:
Volume of carbon monoxide = 100 ml
Mass of carbon monoxide = 0.125 g
Molar volume = 22400 ml
Mass of 1 mole of carbon monoxide =?
Volume : Mass
100 ml : 0.125 g
22400 ml : x g
Mass of one mole of carbon monoxide = 28 g/mole
\ Relative molecular mass of carbon monoxide = 28.
Deduction of relationship between molecular mass and vapour density
Relative Molecular Mass
Relative molecular mass is the ratio of the mass of one molecule of a substance to the mass th of a carbon atom, or 1 amu.
Vapour Density
Vapour density is the ratio of the mass of a volume of a gas, to the mass of an equal volume of hydrogen, measured under the same conditions of temperature and pressure.
According to Avogadro’s law, equal volumes of all gases contain equal number of molecules.
Thus, let the number of molecules in one volume = n,
Cancelling ‘n’ which is common, we get
However, since hydrogen is diatomic.
When we compare the formulae of vapour density with relative molecular molecular mass, they can be represented as
We can therefore substitute the above equation, and arrive at the below formula
Now on cross multiplication, we have
2 x vapour density = Relative Molecular Mass of a gas
or,
Relative Molecular Mass = 2 x Vapour density
MOLE CONCEPT
Since it is not possible to calculate the weight of particles individually, a collection of such particles called mole is taken for all practical purposes. Avogadro discovered that under standard conditions of temperature and pressure, (1atm and 273K) a sample of gas occupies a volume of 22.4 L.
It was discovered that the number of atoms present in 12g of carbon is 6.023 x 1023 atoms. This is referred to as Avogadro number.
A mole of a gas is the amount of a substance containing 6.023 x 1023 particles.
Avogadro’s number is the number of atoms present in C12 isotope = 12g of C.
One mole of any gas at STP will have a volume of 22.4 L.
Mole in term of mass, volume number and ions
In chemistry, the term mole represents a pile or mass of atoms, molecules, ions or electrons. Just as common man measures quantity in terms of kilograms or dozens, a chemical scientist deals with a ‘mole’ of atoms, molecules, ions or electrons.
Mole is defined as the amount of a substance, which contains the same number of chemical units (atoms, molecules, ions or electrons) as there are atoms in exactly 12 grams of pure carbon-12. A mole represents a collection of 6.023 x 1023 ( Avogadro’s number) chemical units. Thus a mole represents the quantity of material, which contains one Avogadro’s number of chemical units of any substance. The unit of mole is denoted as ‘mol’.
For example
One mole of hydrogen atoms = 6.023 x 1023 atoms of hydrogen
One mole of hydrogen molecules = 6.023 x 1023 molecule of hydrogen
One mole of electrons = 6.023 x 1023 electrons
One mole of sodium ions (Na+) = 6.023 x 1023 Na+ ions
It can be thus concluded that,
One mole of atoms = 6.023 x 1023 atoms = Gram atomic mass of the element.
One mole of molecules = 6.023 x 1023 molecules = Gram molecular mass.
Molar Volume
The volume occupied by one mole of any substance is called its molar volume. It is denoted by Vm. Molar volume of the substance depends on temperature and pressure. One mole of all gaseous substances at 273 K and 1 atm pressure occupies a volume equal to 22.4 litre or 22,400 mL. The unit of molar volume is litre per mol or millilitre per mol.
The various relationships of mole can be summarized as follows:
Calculation based on mole concept
Problems
- Calculate the average atomic weight of silicon having 92.2% of Si-28 isotope of relative mass 27.98 amu, 4.7% of Si-29 isotope of relative mass 28.98 amu and 3.1% of Si-30 isotope of relative mass 29.97 amu.
Solution
Data given: 92.20% of silicon of mass 27.98 amu
4.7% of silicon of mass 28.98 amu
3.1 % of silicon of mass 29.97 amu
Average atomic weight
= 25.80 + 1.36 + 0.93
= 28.09 amu.
Average atomic weight of silicon is 28.09 amu.11. One million silver atoms weigh 1.79 x 1016 g. Calculate the gram atomic mass of silver.
Solution
Number of silver atoms = 1 million = 1 x 106
Mass of one million Ag atoms = 1.79 x 1016 g
= 107.8 g
Gram atomic mass of silver is equal to the mass of 6.023 x 1023 atoms of silver. So, the gram atomic mass of silver is 107.8 g
- Chlorophyll, the green colouring matter of plants responsible for photosynthesis, contains 2.68% of magnesium by weight. Calculate the number of Mg atoms in 2.00 g of chlorophyll.
Solution
100 g of chlorophyll contains 2.68 g of Mg
1 mole of Mg = 24 g of Mg = 6.023 x 1023 atom of Mg
24 g of Mg contains, 6.023 x 1023 atom of Mg
=1.34 x 1021 Mg atoms
- A sample of gaseous substance weighing 0.5 g occupies a volume of 1.12 litre under NTP conditions. Calculate the molar mass of the substance.
Solution
1 mole of any gaseous substance at NTP occupies 22.4 L.
1.12 L of gaseous substance = 0.5 g
The molar mass of the substance therefore is 10 g/mol.
REVISION EXERCISES
- If 2g of zinc reacted with excess hydrogen chloride acid, calculate: a .the number of moles of Hydrogen liberated
b . the volume of Hydrogen liberated
(Zn= 63 , 1 mole of any gas at S.T.P=22.4dm3).
- 11.2 dm3 of hydrogen gas was evolved at S.T.P, when magnesium is reacted with excess hydrogen chloride acid. Calculate the mass of magnesium involved in the reaction. Calculate the mass of magnesium involved in the reaction.
WEEK 5
ACID-BASE TITRATION
In acid base titrations, a base is added to an acid successively (or vice versa) and the pH of the solution is noted after every addition.
TITRATION OF STRONG ACID AND STRONG BASE
- The titration of a weak base-alkali with a strong acid
e.g titrating ammonia (pipetted) with standardised hydrochloric acid (in burette) using methyl orange indicator
The apparatus, chemicals and indicator colours are illustrated in the diagram on the left.
Initially the burette is clamped carefully in position and filled with standard hydrochloric acid (e.g. 0.10 to 1.0 mol/dm3, but accurately known, preferably to 4 sig. figs.). The acid is run through until the reading below the meniscus is 0.00 cm3 (the reading in the diagram is 7.00 cm3, which could represent a titration value). The burette is usually calibrated to 50.00 cm3 (only 10.00 cm3 in diagram – couldn’t fit rest of scale on!)
The ammonia solution is accurately measured out into the conical flask with e.g. a 25 cm3 pipette and suction bulb (see diagram further down). Add a few drops of methyl orange indicator to the ammonia solution and it should turn yellow for an alkali. Carefully place the conical flask under the tip of the burette so drops don’t go astray!
The titration: You carefully add small portions of the acid, swirling after each addition and checking the colour of the indicator (not shown in the diagram, but its good to stand the flask on white tile). At the start of the titration the methyl orange indicator is yellow. As you add the acid you get ‘splurges’ of reddish-orange colour until the mixture is swirled in the conical flask. The swirling of the flask contents is important, it ensures all the added hydrochloric acid reacts with the ammonia solution.
Try to add dropwise when you seem to be near the orange colour at the endpoint. The end-point is an orange colour indicating when all the ammonia is neutralised by which ever acid you are using.
If you ‘overshoot’ the titration with excess acid, the methyl orange indicator turns red and the result is invalid. The titration should be repeated several times with other 25 cm3 portions and the average (mean) titration value calculated to use in any subsequent calculations.
How to calculate the concentration of the weak alkali is explained in the top half of the page.
The theory of which indicator to use is explained on the Changes in pH in a neutralisation, choice and use of indicators page.
–
- The titration of a weak/strong a strong acid with sodium hydroxide solution
e.g titrating ethanoic acid or hydrochloric acid (pipetted) with standardised sodium hydroxide solution (in burette) using phenolphthalein indicator
The apparatus, chemicals and indicator colours are illustrated in the diagram on the left.
Initially the burette is clamped carefully in position and filled with standard sodium hydroxide solution (e.g. 0.10 to 1.0 mol/dm3, but accurately known, preferably to 4 sig. figs.). The sodium hydroxide solution is run through until the reading below the meniscus is 0.00 cm3 (the reading in the diagram is 7.00 cm3, which could represent a titration value). The burette is usually calibrated to 50.00 cm3 (only 10.00 cm3 in diagram – couldn’t fit rest of scale on!)
The acid solution is accurately measured out into the conical flask with e.g. a 25 cm3 pipette and suction bulb (see diagram further down). Add a few drops of phenolphthalein indicator to the acid solution and it should turn colourless for an acid. Carefully place the conical flask under the tip of the burette so drops don’t go astray!
The titration: You carefully add small portions of the sodium hydroxide, swirling after each addition and checking the colour of the indicator (not shown in the diagram, but its good to stand the flask on white tile). At the start of the titration the phenolphthalein indicator is colourless. As you add the alkali you get ‘splurges’ of pink colour until the mixture is swirled in the conical flask. The swirling of the flask contents is important, it ensures all the added sodium hydroxide reacts with the acid in the flask.
Try to add dropwise when you seem to be near the faint pink colour of the endpoint. The end-point is the first faint, but permanent pink colour, that is when all the acid is neutralised by the sodium hydroxide.
If you ‘overshoot’ the titration with excess alkali, the phenolphthalein indicator becomes an even deeper pinkish-red and the result is invalid. The titration should be repeated several times with other 25 cm3 portions and the average (mean) titration value calculated to use in any subsequent calculations.
How to calculate the concentration of the weak acid is explained in the top half of the page.
The theory of which indicator to use is explained on the Changes in pH in a neutralisation, choice and use of indicators page.
–
- The titration of a strong base-alkali with hydrochloric solution
e.g titrating sodium hydroxide solution (pipetted) with standardised hydrochloric solution (in burette) using phenolphthalein indicator
The apparatus, chemicals and indicator colours are illustrated in the diagram on the left.
Initially the burette is clamped carefully in position and filled with standard hydrochloric acid (e.g. 0.10 to 1.0 mol/dm3, but accurately known, preferably to 4 sig. figs.). The hydrochloric acid is run through until the reading below the meniscus is 0.00 cm3 (the reading in the diagram is 7.00 cm3, which could represent a titration value). The burette is usually calibrated to 50.00 cm3 (only 10.00 cm3 in diagram – couldn’t fit rest of scale on!)
The alkali solution is accurately measured out into the conical flask with e.g. a 25 cm3 pipette and suction bulb (see diagram further down). Add a few drops of phenolphthalein indicator to the alkali solution and it should turn deep pink for an alkali. Carefully place the conical flask under the tip of the burette so drops don’t go astray!
The titration: You carefully add small portions of the acid, swirling after each addition and checking the colour of the indicator (not shown in the diagram, but its good to stand the flask on white tile). At the start of the titration the phenolphthalein indicator is a deep reddish-pink in alkaline solution. As you add the acid you get ‘splurges’ of colourless solution until the mixture is swirled in the conical flask. The swirling of the flask contents is important, it ensures all the added hydrochloric acid reacts with the sodium hydroxide.
Try to add dropwise when you seem to be near the faintest pinkness left in the solution near the endpoint.
The end-point is when the last trace of pink colour first disappears from the solution. If you ‘overshoot’ the titration with excess acid, it still stays colourless and the result is invalid. The titration should be repeated several times with other 25 cm3 portions and the average (mean) titration value calculated to use in any subsequent calculations
–
Selection of indicators in acid-base titration and PH curve
Selection of indicators in acid-base titration
The amount of an acid (or a base), which is exactly equivalent chemically to the amount of some standard base (or an acid), is determined by an acid-base titration. The point of equivalence is called end point. The solution of a strong acid and strong base will be neutral at the end point and have a pH of 7 as they are strong electrolytes. However, if either the acid or the base is a weak electrolyte, the solution at the equivalence point will be either slightly alkaline (pH>7) or slightly acidic (pH<7).>+ ion concentration (i.e. pH), which depends upon the nature of the acid and the base and the concentration in the solution.
A large number of acid-base indicators are available which possess different colours according to H+ion concentration of the solution. These indicators do not change from predominantly ‘acid’ colour to predominantly ‘base’ suddenly and abruptly, but within a small interval of pH (generally about 2 pH units). This is called colour-change interval of the indicator. The position of the colour-change interval in the pH scale varies widely with different indicators. Therefore, for acid base titrations, an indicator is chosen which shows a distinct colour change at a pH close to that of equivalent point.
Colour changes of indicators with pH
Indicator pH range Colour Acid solution Colour base solution
Cresol blue 1.2 — 1.8 Red Yellow
Thymol blue 1.2 — 2.8 Red Yellow
Methyl yellow 2.9 — 4.0 Red Yellow
Methyl orange 3.1 — 4.4 Pink Yellow
Methyl red 4.2 — 6.3 Red Yellow
Litmus 5.0 — 8.0 Red Blue
Bromothymol blue 6.0 — 7.6 Yellow Blue
Phenol red 6.4 —8.2 Yellow Red
Thymol blue (base) 8.1 — 9.6 Yellow Blue
Phenolphthalein 8.3 — 10.0 Colourless Blue
Thymolphthalein 8.3 — 10.5 Colourless Blue
Alizarin yellow R 10.1 — 12.0 Blue Yellow
Nitrarnine 10.0 — 13.0 Colourless Orange/brown
REVISION EXERCISES
5.00g of Na O H were dissolved in 1000cm3. 25cm3 of this solution neutralized 28.30cm3 of solution containing 7.20gdm3 of impure tetraoxosulphate (vi) solution, calculate
i . molar concentration of acid
- mass concentration of acid
iii. the percentage impurity of acid
(H=1.0, O=16, Na=23)
WEEK 6
INTRODUCTION TO ORGANIC CHEMISTRY
Organic chemistry is that branch of chemistry which deals with the study of compounds of carbon with hydrogen (hydrocarbons), and their derivatives. Presently about five million organic compounds are known.
Organic compounds
The compounds of carbon generally containing carbon-carbon bonds are called organic compounds. These occur in carbon compounds with hydrogen and their derivatives. For thyl alcohol, sugar, starch are organic compounds.
Origin of organic compounds
Carbon compounds are of two types: inorganic and organic. The compounds that have a mineral origin fall under the category of inorganic compounds. The compounds having plant or animal origin are classified as organic compounds. Lavoisier showed that nearly all compounds of plant origin are composed of carbon, hydrogen and oxygen. While those of animal origin also had other substances like nitrogen, sulphur or phosphorus. In spite of the fact that organic compounds were originally derived from living sources, today, most of these compounds can be synthesized.
Organic compounds were found to contain mainly hydrogen and carbon. Therefore, organic chemistry is defined as the study of hydrocarbons and their derivatives.
Reasons for studying organic compounds as a separate branch of chemistry
Organic compounds are studied as a separate branch of chemistry because of the following reasons:
- Existence of innumerable organic compounds.
- Properties of organic compounds are distinctly different from those of inorganic compounds.
Study of organic chemistry is important for the simple reason that, organic compounds find applications in almost all aspects of our daily life.
The list given below illustrates the importance of organic compounds in our daily life:
Application of Organic Compounds in Daily Life
- Foods [starch, sugar, fats, vitamins, proteins]
- Fuels [wood, coal, alcohol, petrol]
- Household and commercial articles [paper, soap, cosmetics, oils, paints]
- Textile fabrics [cotton, wool, silk, linen, rayon, nylon]
- Drugs and disinfectants [penicillin, quinine, aspirin, sulpha drugs]
- Poisons [opium, strychnine]
- Perfumes [vanillin, camphor]
- Explosives [nitroglycerine, dynamite, picric acid, TNT]
- Dyes [indigo, congo red, malachite green]
- War gases [mustard gas, chloropicrin, lewisite]
Tetra covalency and catenation property of carbon
Tracovalency
A carbon atom has a total of six electrons occupying the first two shells, i.e., the K-shell has two electrons and the L-shell has four electrons. This distribution indicates that in the outermost shell there are one completely filled ‘s’ orbital and two half-filled ‘p’ orbitals, showing carbon to be a divalent atom. But in actuality, carbon displays tetravalency in the combined state. Therefore, a carbon atom has four valence electrons. It could gain four electrons to form C4- anion or lose four electrons to form C4+ cation. Both these conditions would take carbon far away from achieving stability by the octect rule. To overcome this problem carbon undergoes bonding by sharing its valence electrons. This allows it to be covalently bonded to one, two, three or four carbon atoms or atoms of other elements or groups of atoms. Let us see how carbon forms the single, double and triple bonds in the following examples.
Methane Molecule
Carbon atom has four electrons in its outermost shell. Thus, it requires four more electrons to acquire a stable noble gas configuration. Each of the hydrogen atoms has only one electron in its outermost shell and requires one more electron to complete its outermost shell (to acquire He configuration). This is done as follows.
Carbon Dioxide Molecule
The electronic configurations of carbon and oxygen are:
Thus, each carbon atom requires four, and each oxygen atom requires two more electrons to acquire noble gas configurations. To achieve this, two oxygen atoms form a double covalent bond with carbon as follows.
Acetylene Molecule
Carbon atom has four electrons in its outermost shell and hydrogen atoms have only one electron in its outermost shell. Carbon share one of its electrons with hydrogen to form a single bond each. Each carbon then requires three more electrons to acquire a stable configuration of the nearest noble gas (neon). This is done by mutually sharing three pairs of electrons between the two carbon atoms to form a triple bond as shown below.
Catenation
The property of self-linking with atoms of the same element is termed Catenation. Carbon has a unique property of linking itself to other carbon atoms to give open chain or/and cyclic structures. Catenation is favored by atoms where atom to atom covalent bond is quite strong.
In carbon, C-C bond energy is very high (347.3 kJ mol-1) causing catenation. Further, the carbon atom due to its tetravalency, can be bonded to two, three or four carbon atoms by forming single and multiple bonds. Therefore, chains of carbon atoms may be linear, branched or cyclic. For example,
Catenation is responsible for the existence of a large number of organic compounds.
Strength of Bonds with Other Elements
The C-C and C-H bonds in organic compounds are very strong. During chemical reactions, these bonds generally do not break easily. However other atoms or groups such as Cl, OH, etc., attached to the carbon atom may get replaced easily. For example, aqueous KOH reacts with haloalkanes to give the corresponding alcohol
All these features make carbon to form a mole variety of compounds
Classification of organic compounds
There are millions of organic compounds and this makes it physically impossible to study each individual compound. To facilitate their study, organic compounds are classified into various groups and sub-groups.
They may be broadly classified into the following classes:
- Open chain or acyclic compounds.
- Closed chain or cyclic (or ring) compounds.
These compounds contain an open chain system of carbon atoms. The chains may be either straight chains (non-branched) or branched. The open chain compounds are also called aliphatic compounds. The name aliphatic is derived from the Greek word aleiphar meaning fats, as the earlier known compounds of this class were either obtained from animal or vegetable fats, or had fat like character.
Straight chain (or non-branched) compounds
CH3-CH2-CH2-CH3 CH3-CH2-CH2-CH2OH H2C = CH2
Butane 1- butanol ethene
Branched chain compounds
These compounds contain one or more closed chains (rings) of atoms and are known as cyclic or ring compounds.
These are of two types:
- Homocyclic compounds, or carbocyclic compounds
- Heterocyclic compounds.
Homocyclic or Carbocyclic compounds
The compounds in which the ring consists of only carbon atoms are called homocyclic or carbocyclic compounds. Homocyclic compounds or carbocylic compounds are further divided into:
(a) Alicyclic compounds (b) Aromatic compounds
Alicyclic compounds
When a ring of three or more carbon atoms resembling aliphatic compounds are contained in homocyclic or carbocyclic compounds, they are called alicyclic compounds. The saturated alicyclic hydrocarbons have the general formula CnH2n. Typical alicyclic compounds are given below.
cyclopropane cyclobutane cyclohexane
cyclopropene cyclohexene cyclohexa-1,4-diene cyclopentene
Aromatic compounds
These carbocylic compounds contain at east one benzene ring i.e., a ring of six carbons atoms with alternate double and single bonds. Aromatic compounds are called so because many of them possess a fragrant smell.
Typical examples of aromatic compounds are given below:
Benzene
The aromatic compounds may have a side-chain or a functional group attached directly to the ring. For example,
Toluene Phenol Nitrobenzene Benzaldehyde
The aromatic compounds may also contain more than one benzene rings fused together
Naphthalene Anthracene
Heterocyclic compounds
When atoms of more than one kind make up the ring in the compounds, they are known as heterocyclic compounds or heterocycles. In these compounds generally one or more atoms of elements such as nitrogen ‘N’, oxygen ‘O’, or sulphur ‘S’ are present. The atom other than that of carbon viz., N, O or S, present in the ring is called hetero atom. Heterocyclic compounds with five and six atoms in the ring are termed as five-membered, and six-membered heterocycles respectively.
Pyridine Furan Thiophene Pyrrole
Heterocyclic compounds may be further classified as monocyclic, bicyclic and tricyclic depending on the presence of one, two or three rings in their molecules, respectively.
Compounds, which contain nitrogen atom ‘N’ in their ring, have names usually ending with ‘ole’, if five-membered and with ‘inc’, if six-membered. Hetero atom is always numbered as atom number 1, (except in one or two cases).
Hydrocarbons can be further classified into four types on the basis of their structures. These are:
Alkanes
Hydrocarbons that contain only C-C single bonds in their molecules are called alkanes. These include open chain as well as closed chain (cyclic) hydrocarbons. For example, ethane, propane cyclopentane.
Alkanes are further divided into:
- Open chain or acyclic (simple alkanes not having any closed chains). They have the general formula CnH2n+2. Examples are methane(CH4), propane(C3H8) and butane(C4H10).
- Cycloalkanes or cyclic alkanes (having a closed chain or rings in their molecules). They have the general formula CnH2n. Examples are cyclopropane(C3H6) and cyclobutane(C4H8).
Alkenes
These are hydrocarbons that contain at least one carbon-carbon double bond. For example, ethene, but-2-ene, but-1-ene.
Alkynes
These hydrocarbons contain at least one carbon-carbon triple bond. For example, ethyne, propyne.
Arenes
These are hydrocarbons that contain at least one special type of hexagonal ring of carbon atoms with three double bonds in their alternate positions. The ring is called aromatic or benzene ring.
For example, benzene, toluene, o-xylene. They also contain more than one benzene rings. For example, naphthalene (2 rings) and anthracene (3 rings).
Hydrocarbons can also be classified into:
- Saturated hydrocarbons
Those that contain carbon-carbon single bonds e.g. alkanes(C-C).
- Unsaturated hydrocarbons
Those that contain carbon-carbon double or triple bonds e.g. alkenes(C=C), alkynes(C=C).
Functional groups and homologous series
Functional groups
When an atom or group of atoms bonded to a carbon atom in the chain or ring of an organic compound, shown some characteristic properties of their own, they are termed as a functional group. Thus, a functional group is an atom or group of atoms which determine the chemical behavior of an organic compound. All the compounds having a particular functional group behave alike. For example all compounds containing -OH (hydroxyl) group undergo similar reactions.
The functional group present in the following molecules are encircled.
Common functional groups and their families of compound
Homologous series
A homologous series is a family of organic compounds containing a particular characteristic group and exhibiting similar properties. For example, the compounds given below belong to the alcohol family.
CH3OH methyl alcohol (methanol)
CH3CH2CH2OH propyl alcohol (1-propanol)
CH3CH2.CH2.CH2OH butyl alcohol (1-butanol)
Characteristics of a Homologous Series
All members of a homologous series exhibit some common characteristics. They are:
- All the members of a homologous series can be represented by a common general formula, as they have the same functional group. For example, alkanes can be represented by the formula CnH2n+2.
CH4 C2H5 C3H8 C4H10
Methane Ethane Propane Butane
- Each member of a homologous series has a common difference of -CH2 from the next higher or lower member.
- Common general methods of preparation exist for all members of the series.
- All members exhibit similar chemical behavior.
- An increase in molecular mass of members within a homologous series show a similar regular gradation of the physical properties, such as, physical state, melting and boiling points etc.
Meaning of empirical formula, molecular formula, structural formula and contracted formula
In chemistry, the empirical formula of a chemical compound is the simplest whole number ratio ofatoms of each element present in a compound.[1] An empirical formula makes no reference toisomerism, structure, or absolute number of atoms. The empirical formula is used as standard for most ionic compounds, such as CaCl2, and for macromolecules, such as SiO2. The term empirical refers to the process of elemental analysis, a technique of analytical chemistry used to determine the relative amounts of each element in a chemical compound.
In contrast, the molecular formula identifies the number of each type of atom in a molecule, and thestructural formula also shows the structure of the molecule.
For example, the chemical compound n-hexane has the structural formula CH3CH2CH2CH2CH2CH3, which shows that it has 6 carbon atoms arranged in a straight chain, and 14 hydrogen atoms. Hexane’s molecular formula is C6H14, and its empirical formula is C3H7, showing a C:H ratio of 3:7. Different compounds can have the same empirical formula. For example, formaldehyde, acetic acid and glucosehave the same empirical formula, CH2O. This is the actual chemical formula for formaldehyde, but acetic acid has double the number of atoms and glucose has six times the number of atoms.
A chemical formula or molecular formula is a way of expressing information about the atoms that constitute a particular chemical compound.
The chemical formula identifies each constituent element by its chemical symboland indicates the number of atoms of each element found in each discretemolecule of that compound. If a molecule contains more than one atom of a particular element, this quantity is indicated using a subscript after the chemical symbol (although 18th-century books often used superscripts) and also can be combined by more chemical elements.
Chemical formulas may be used in chemical equations to describe chemical reactions.
For ionic compounds and other non-molecular substances an empirical formula may be used, in which the subscripts indicate the ratio of the elements.
The 19th-century Swedish chemist Jöns Jakob Berzelius worked out this system for writing chemical formulas.
The structural formula of a chemical compound is a graphical representation of the molecular structure, showing how the atoms are arranged. The chemical bonding within the molecule is also shown, either explicitly or implicitly. There are three common representations used in publications: text, Lewis typeand line-angle formula. Also several other formats are used, as in chemical databases, such as SMILES,InChI and CML.
Unlike chemical formulae or chemical names, structural formulas provide a representation of the molecular structure. Chemists nearly always describe a chemical reaction or synthesis using structural formulae rather than chemical names, because the structural formulas allow the chemist to visualize the molecules and the changes that occur.
Many chemical compounds exist in different isomeric forms, which have different structures but the same overall chemical formula. A structural formula indicates the arrangements of atoms in a way that a chemical formula cannot.
Common names of organic compounds
Common nomenclature
In the common system, cyanides are named by any of the following methods:
(i) By using suffix cyanide after the name of alkyl and aryl group.
or
(ii) By adding the suffix o-nitrile in place of ic – acid in the common name of the corresponding acid produced by the hydrolysis of the cyanide compound.
For e.g., CH3 CN on hydrolysis gives CH3 COOH i.e., acetic acid.
So the common name of the compound is methyl cyanide or Acetonitrile as derived from acetic acid.
IUPAC nomenclature
In the IUPAC system, suffix nitrile is added to the name of the hydrocarbon atoms ie they are named as Alkanenitriles. The carbon atom of the -CN group is also counted in the parent chain. For determining the position of the substituent in a chain, the nitrile carbon is numbered as 1.
Example:
IUPAC system and IUPAC rules of naming hydrocarbon
Nomenclature is the system of naming. With the existence of innumerable compounds of carbon, it has become necessary to follow a universal, rational system of naming. Such a system has been evolved by International union of pure and applied chemistry (IUPAC). By following this system, we can eliminate the confusion that arises due to usage of common names or trivial names.
Nomenclature of straight chain hydrocarbons
The IUPAC name of a straight chain hydrocarbon is divided into two parts:
- Word root
- Suffix
Word root
Word root determines the number of carbon atoms in the compound. The word roots for compounds having five or more carbon atoms are derived from Greek numbers.
Chain length C1 C2 C3 C4 C5 C6 C7 C8 C9 C10
Word root Meth – Eth – Prop – But – Pent – Hex – Hept – Oct – Non – Dec –
Suffix
To determine the nature of the bond (single, double or triple) between the carbon atoms, primary suffixes are used.
Hydrocarbon Primary suffix General name
Saturated C – C – ane Alkane
Unsaturated C = C – ene Alkene
Unsaturated C ≡ C – yne Alkyne
Nomenclature of saturated hydrocarbons (alkanes)
In the IUPAC nomenculature, alkanes end with ‘-ane’
Condensed Formula Word root Primary suffix IUPAC name and Formula
- CH4
- CH3CH3
- CH3CH2CH3
- CH3CH2CH2CH3
- CH3CH2CH2CH2CH3 Meth –
Eth –
Prop –
But –
Pent – ane
- ane
ane
-ane
- ane Methane CH4
Ethane C2H6
Propane C3H8
Butane C4H10
Pentane C5H12
Nomenclature of unsaturated hydrocarbons
Alkenes
In the IUPAC nomenclature, alkenes end with ‘-ene’
Condensed Formula Eord root primary suffix IUPAC name and Formula
- CH2 = CH2 Eth – – ene Ethene C2H2
2.CH3 – CH = CH2 Prop – – ene Propene C3H6
3.CH3 – CH2 – CH = CH2 But – – ene Butene C4H8
4.CH3 – CH2 – CH – = CH2 pent – – ene Pentene C5H10
Alkynes
In the IUPAC nomenculature, alkenes end with ‘-yne’.
Condensed Formula Word root Primary Suffix IUPAC name and formula
CH ≡ CH Eth – – yne Ethyne C2H2
CH3 – C ≡ CH Prop – – yne Propyne C3H4
CH3 – CH2 – C ≡ CH But – – yne Butyne C4H6
CH3 – CH2 – CH2 – C ≡ CH Pent – – yne Pentyne C5H8
Definition of structure isomerism
When two or more organic compounds have the same molecular formula but different structural formulae, they exhibit structural isomerism.
There are 5 different kinds of structural isomerism. They are:
- chain or nuclear isomerism
- position isomerism
- functional isomerism
- metamerism
- tautomerism
Types of structure isomerism
Chain or nuclear isomerism
Two organic compounds having same molecular formula differ in the nature of the carbon atom chain. This kind of isomerism is known as chain or nuclear isomerism. For e.g., n-butane and isobutane, have the same molecular formula C4H10. While n-butane is a straight chain, isobutane has a branched structure.
Position isomerism
When the same substituent occupies different positions in organic compounds having same molecular formula, the compounds exhibit position isomerism. For e.g.,
H3C-CH2-CH2-I is n-propyl iodide or 1-iodopropane and H3C-CHI-CH3 is isopropyl iodide or 2-iodopropane.
Note that both the compounds have the same molecular formula C3H7I. In n-propyl iodide the substituent is attached to the first carbon atom whereas in isopropyl iodide it is attached to the second carbon atom.
Functional isomerism
Organic compounds having same molecular formula but different functional groups exhibit functional isomerism. For e.g., ethanol (C2H5OH) and methoxy methane (H3C-O-CH3) have the same molecular formula C3H6O. But the functional group in ethanol is the alcohol group whereas in methoxy methane, it is the ether group.
Metamerism
Some members of the same homologous series differ in the nature of the alkyl groups attached to the polyvalent atom of the functional group. Such compounds are said to exhibit metamerism.
For e.g., diethyl ether (H5C2OC2H5), methyl n-propyl ether (H3COCH2CH2H3C) and methyl isopropyl ether [H3COCH(CH3)2]are metamers. All of them have the same molecular formula C4H10O but the alkyl groups are different.
Tautomerism
Tautomerism is a dynamic isomerism where one isomer is constantly changing into the other and vice versa. For e.g., alky cyanides (RCN) and alkyl isocyanides (RNC) are tautomers.
REVISION EXERCISES
- The empirical formula of a compound which has molecular formula of C4H8N2O2 is——-
- The general molecular formular of alkenes is——–
3 .W hat are Hydrocarbons?
- List three types.
- What is an homologous series? Give three characteristics of an homologous series.
ALKANES
WEEK 7
What are Alkanes
Alkanes contain only Carbon and hydrogen atoms. They have only single bonds between Carbon atoms (C-C bonds). They are called “saturated hydrocarbons.” Organic molecules that are formed with only Carbon and Hydrogen atoms are called “hydrocarbons.” According to the orbital hybridization model, all the carbon atoms in Alkanes have the SP3 hybridization. They form sigma bonds with Hydrogen atoms resulting the molecular geometry like a tetrahedron.
General molecular formula of Alkanes
Alkanes have the general molecular formula CnH2n+2. The smallest alkane is Methane (CH4).
Molecular structure of Alkanes
Acyclic alkanes: There is no ring formation in the structure. However, it can have branched or unbranched molecular arrangements. Unbranched Alkanes are sometimes called n-alkanes.
Cycloalkanes: There is a circular molecular arrangement in the structure. Cycloalkanes have the general formula CnH2n.
Chemical properties of Alkanes
Reactivity
Alkanes are inert to many chemical reagents. “Paraffin” is an old name for hydrocarbons. It is derived from the Latin word “parumaffinis,” which means “with little affinity”. The reason is Carbon–Carbon (C-C) and Carbon–Hydrogen (C-H) bonds are quite strong. It is very difficult to break their bonds unless alkanes are heated to fairly high temperatures. The C-H bonds are also strong, because Carbon and Hydrogen atoms have nearly the same electronegativity values.
Combustion
Alkanes can readily burn in the air. Reaction between Alkanes with excess Oxygen is called “combustion.” In this reaction, alkanes convert to Carbon dioxide (CO2) and water.
The combustion reactions are exothermic, which means they give off heat. Therefore, alkanes can be used as a source of energy.
Physical Properties of Alkanes
Alkanes exist in all three forms: as gases, liquids and solids. Methane, Ethane, propane, and butane are gases in room temperature. The unbranched structures of pentane, hexane, and heptane are liquids. Alkanes with higher molecular weight are solids.
CH4 C4H10 Gases
C5H12 C17H36 Liquids
Alkanes with higher molecular weight Soft solids
Solubility
Alkanes are a non-polar organic compound. Water is a polar solvent, so alkanes do not dissolve in water. They are said to be “hydrophobic” (means ‘water hating’) compounds. They are dissolved in non-polar or weakly polar organic solvents. Alkanes are used as good lubricants and preservatives for the metals because, they protect the metal surface from reaching water; it prevents corrosion.
Density
The densities of Alkanes are lower than the density of water. Their density value is nearly 0.7 g mL-1, considering the density of water as 1.0 g mL-1. For example, if we mix an Alkane with water, the Alkane layer separates on the top of the water, since Alkanes are less dense compared to water and they are insoluble in water.
Boiling points
For unbranched alkanes, the boiling point smoothly increases as the number of Carbon atoms and the molecular weight are increasing. Larger molecules have a larger surface area providing a greater ability to form van der waals interactions (London force interactions). Though these are weak intermolecular forces, they raise the boiling points and thus prevent vapourisation.
In general, branched alkanes have lower boiling points compared to the same unbranched alkanes, having the same number of Carbon atoms. The differences in boiling points arise since branched alkanes are more compact is a small surface area, and thus facilitating less surface area for London force interactions. This lowers the boiling points in branched alkanes.
Melting points
For n-alkanes, this follows the same variation as the melting points; melting point increases with the molecular weight. However, there is a slight difference in melting points between the alkanes with even number of Carbon atoms and odd number of Carbon atoms. Alkanes with even number of Carbon atoms have higher melting points, because they are packed well into a solid structure. Therefore, a higher temperature along the alkane series is required to melt them. Therefore, the variation of melting points does not show a smooth curve along the alkane series.
In general, branched alkanes have higher melting points than n-alkane with the same number of Carbon atoms. Branched structure gives a more compact 3D-structure. It easily packs into a solid structure with a high melting point.
.
The first four gaseous alkanes are very flammable and explosive in air!
n molecular formula (state at RTP) abbreviated structural formula name of alkane relative molecular mass Mr melting point temperature oC/K boiling point temperature oC/K flash point oC density g/cm3
1 CH4 (g) CH4 methane 16.0 –182/91 –164/109 na 0.466(l)
2 C2H6 (g) CH3CH3 ethane 30.1 –183/90 –88/185 na 0.572(l)
3 C3H8 (g) CH3CH2CH3 propane 44.1 –190/83 –42/231 na 0.585(l)
4 C4H10 (g) CH3CH2CH2CH3 butane 58.1 –138/135 0/273 na 0.601(l)
5 C5H12 (l) CH3(CH2)4CH3 pentane 72.2 –130/143 36/309 –49 0.626
6 C6H14 (l) CH3(CH2)4CH3 hexane 86.2 –95/178 69/342 –22 0.660
7 C7H16 (l) CH3(CH2)5CH3 heptane 100.2 –90/183 99/372 –4 0.684
8 C8H18 (l) CH3(CH2)6CH3 octane 114.2 –57/216 126/399 13 0.703
9 C9H20 (l) CH3(CH2)7CH3 nonane 128.3 –51/222 151/424 31 0.718
10 C10H22 (l) CH3(CH2)8CH3 decane 142.3 –30/243 174/447 46 0.730
11 C11H24 (l) CH3(CH2)9CH3 undecane 156.3 –25/248 196/469 60 0.740
12 C12H26 (l) CH3(CH2)10CH3 dodecane 170.3 –9/264 216/489 71 0.749
13 C13H28 (l) CH3(CH2)11CH3 tridecane 184.4 –5/268 234/507 102 0.756
14 C14H30 (l) CH3(CH2)12CH3 tetradecane 198.4 4/279 250/523 99 0.763
15 C15H32 (l) CH3(CH2)13CH3 pentadecane 212.4 10/283 267/540 132 0.769
16 C16H34 (l) CH3(CH2)14CH3 hexadecane 226.4 18/291 281/554 135 0.773
17 C17H36 (l) CH3(CH2)15CH3 heptadecane 240.5 22/295 302/575 148 0.777
18 C18H38 (s) CH3(CH2)16CH3 octadecane 254.5 28/301 326/599 165 0.777
19 C19H40 (s) CH3(CH2)17CH3 nonadecane 268.5 31/304 330/603 168 0.786
20 C20H42 (s) CH3(CH2)18CH3 eicosane 282.5 37/310 343/616 na 0.789
n molecular formula abbreviated structural formula name of alkane relative molecular mass Mr melting point temperature oC/K boiling point temperature oC/K flash point oC density g/cm3
Note: (i) RTP = room temperature and pressure, (ii) na = not applicable to that alkane, (III) the use of parentheses (brackets) to give, for long molecules like the higher alkanes, a more convenient abbreviated formula.
e.g. CH3CH2CH2CH2CH2CH2CH3 can be expressed as CH3(CH2)5CH3
Table showing the structure of the first twelve ALKANES name of alkane
(1=2) (3a) (3b)
(4a) (4b) methane
(main molecule in natural gas)
(1) (2) (3a) (3b)
(4a) (4b)
(5) you can’t see the 6th H atom! ethane
(1) (2)
(3a) (3b) (4a) propane
in bottled gas
(1) (2)
(3a) (3b) butane
in bottled gas
The full displayed formula for the first five members of the homologous series of ALKANES
These diagrams show ALL the covalent bonds (C-H and C-C) in alkane molecules
The formulae can also be written as: CH4, CH3CH3, CH3CH2CH3, CH3CH2CH2CH3 and CH3CH2CH2CH2CH3
The final examples are shown as the displayed formula and molecular formula of the alkane
C5H12
C6H14
C7H16 pentane, hexane and heptane in petrol
C8H18
C9H20 octane and nonane
C10H22
C11H24
C12H26
NOTE: Although the longer alkanes are drawn above in a linear way, in reality, the molecule is very flexible and can adopt all sorts of ‘wiggly’ shapes. See the diagram below as examples of the multitude of shapes the alkane molecules can adopt! The backbone of carbon atoms of the alkane molecules are quite flexible and the longer the chain the more flexible or ‘wiggly’ they are! decane, undecane and dodecane
There are hundreds of different alkanes known and many do not have a ‘straight’ chain of carbon atoms
but have ‘branches’, some are shown below, but don’t bother about their names (leave that for A level!)
this last one is called ‘isooctane’ and is an ingredient of petrol to ensure smoother combustion in car engines.
chemical properties of alkanes
Alkanes are quite inert substances with highly stable nature. Their inactiveness has been explained as:
(i) In alkanes all the C-C & C-H bonds being stonger sigma bonds and are not influenced by acids, alkalies, oxidants under ordinary conditions.
(ii) The C-C (completely non polar) & C-H (weak polar) bonds in alkanes- are practically non polar because of small electronegativity difference in C (2.6) and H (2.1). Thus polar species i.e., electrophiles or nucleophiles are unable to attack these bonds under ordinary conditions.
Inspite of less reactive nature, alkanes show some characteristic reactions.
(1) Oxidation: Oxidation of alkanes gives different products under different conditions.
(a) Complete oxidation or combustion : Alkanes burn readily with non luminous flame in presence of air or oxygen to give CO2 & water along with evolution of heat. Therefore alkanes are used as fuels.
CnH2n+2 + [(3n+1)/2]O2 → nCO2 + (n+1)H2O; ΔH = -ve
CH4+ 2O2 → CO2 + 2H2O; ΔH = -ve
(b) Incomplete oxidation : Incomplete oxidation of alkanes in limited supply of air gives carbon black and carbon monoxide.
2CH4 + 3O2 → 2CO + 4H2O
CH4 + O2 → C + 2H2O
carbon black
(c) Catalytic oxidation :
(i) Lower alkanes are easily converted to alcohols and aldehydes under controlled catalytic oxidation.
(ii) Higher alkanes on oxidation in presence of manganese acetate give fatty acids.
CH3(CH2)nCH3 + 3O2 CH3(CH2)nCOOH
(d) Chemical oxidation : Tertiary alkanes are oxidized to tertiary alcohols by KMnO4
- Substitution reactions :
(i) Substitution in alkanes shows free radical mechanism. For mechanism see free radical substitution.
(ii) Following substitution reactions in alkanes are noticed.
(a) Halogenation :
(i) Replacement of H atom of alkane by halogen atom is known as halogenations.
(ii) Halogenation of alkane is made on exposure to
halogen + alkane mixture to ultraviolet light or at elevated temperature.
Methane and chlorine
Substitution reactions happen in which hydrogen atoms in the methane are replaced one at a time by chlorine atoms. You end up with a mixture of chloromethane, dichloromethane, trichloromethane and tetrachloromethane.
R-H + X2 R-X + HX
(iii) The extent of halogenations depends upon the amount of halogen used.
(iv) The reactivity order for halogens shows the
order : F2 > CI2 > Br2 > I2
(v) F2 reacts violently even in dark and reaction may be controlled by diluting fluorine with N2, whereas iodination is very slow and reversible. Therefore iodination is made in presence of HgO or HIO3 (oxidants which decompose HI)
[CH4 + I2 ↔ CH3I + HI] × 5
5HI + HIO3 → 3I2 + 3H2O
4CH4 + 2I2 + HIO3 → 5CH3I + 3H2O
(vi) The reactivity order for H atom in alkane shows the order:
tertiary hydrogen>secondary hydrogen>primary hydrogen> CH4
(vii) The halogenations is catalysed by dibenzoyl peroxide.
(b) Nitration :
(i) Replacement of H atom of alkane by -NO2 group is known as nitration.
(ii) Nitration of alkane is made by heating vapours of alkanes and HNO3 at about 400oC to give nitroalkanes. This is also known as vapour phase nitration.
CH4(g) + HNO3(g) CH3NO2 + H2O
(iii) During nitration, C-C bonds of alkanes are also decomposed due to strong oxidant nature of HNO3 to produce all possible nitroalkanes.
(iv) The nitration of alkane also shows the order:
T.H. > S.H. > P.H. > methane
(v) The nitration of alkanes follows free-radical mechanism
HONO2 HO + NO2
C3H7-H + HO → C3H7 + H2O
C3H7 + NO2 → C3H7NO2
(c) Sulphonation :
(i) Replacement of H atom of alkane by -SO3H is known as sulphonation.
(ii) Lower normal alkanes are not suphonated, but higher normal alkanes show sulphonation (hexane onwards) when heated with oleum (i.e., conc. H2SO4) at 400oC.
C6H14 + H2SO4 → C6H13SO3H + H2O
(iii) Lower members are sulphonated in vapour phase sulphonation.
(iv) The reactivity order for sulphonation is T.H. > S.H. > P.H. Thus isobutene is easily sulphonated as it contains tertiary hydrogen atom.
(v) Sulphonation of alkanes also follows free radical mechanism.
HOSO3H HO + SO3H
C3H13-H + OH → C6H13 + H2O
C3H13 + SO2H → C6H13SO3H
- Isomerization :
(i) The process of conversion of one isomer into other is known isomerization.
(ii) Straight chain alkanes on heating with AICI3 + HCI at about 200oC and 35 atm pressure are converted into branched chain alkanes.
- Aromatization :
(i) The process of conversion of aliphatic compound into aromatic compound is known as aromatization.
(ii) Alkanes having six to 10 carbon atoms are converted into benzene and its homologues at high pressure and temperature in presence of catalyst.
C6H14 + 4H2
- Pyrolysis :
(i) The decomposition of a compound on heating in absence of air is known as pyrolysis.
(ii) The phenomenon of pyrolysis of alkane is also known as cracking.
(iii) Alkane vapours on passing through red hot metal tube in absence of air decomposes to simpler hydrocarbons. The product formed during cracking depends upon
(a) nature of alkane
(b) temperature and pressure
(c) presence or absence of catalyst
(iv) The ease of cracking in alkanes increases with increase in molecular weight and branching in alkane.
(v) Fission of C-C bonds produces alkane and alkenes whereas fission of C-H bonds produces alkene and hydrogen.
(vi) Presence of Cr2O3, V2O2, MoO3 catalyses C-H bond fission and presence of SiO2, AI2O3, ZnO catalyses C-C bond fission.
(vii) The no. of products obtained during cracking increases with increase in molecular weight of alkane undergoing cracking.
(viii)
(ix) Cracking has an important role in petroleum industry. Higher alkanes are converted into lower one (petrol C6 to C11) by cracking.
- Dehydrogenation : Alkanes are dehydrogenated on heating in presence of catalyst to produce corresponding alkenes.
C3H8 C3H6 + H2
General methods of preparation of alkanes
General Method of Preparation of Alkanes (Paraffins):
Alkanes can be prepared by the following methods:
- From unsaturated Hydrocarbons
- From Haloalkanes
- By Wurtz Reaction
- By reduction
- By the Reduction of Aldehydes and Ketones
- From Grignard’s Reagent
- From salts of Carboxylic acids
- By Kolbe’s electrolytic method
- By Heating Na-salt of Carboxylic acid
- From Metal Carbides
- From unsaturated Hydrocarbons
Alkanes can be prepared by the catalytic hydrogenation of unsaturated hydrocarbons in the presence of catalyst ‘Ni’ or ‘pt’ at 200⁰C to 300⁰C.
- From Haloalkanes
- By Wurtz Reaction:
When alkyl halides are heated with sodium metal in the presence of dry ether, alkanes are obtained (generally having double number of C-atoms than in alkyl halides). This reaction is known as Wurtz reaction and used for the preparation of symmetrical alkanes.
- By Reduction of alkyl halides (RX)
Haloalkanes (R-X) when heated with reducing agents like; LiAlH4/ether, Pd/H2, Pt/H2, Zn/conc. HCl, alkanes are produced.
R-X R-H + HX
- By the Reduction of Aldehydes and Ketones
Aldehydes and ketones can be reduced into alkanes in the presence of reducing agents: amalgated zinc and conc. HCl.
- From Grignard’s Reagent
Hydrolysis of Grignard’s reagent in the presence of ether gives alkanes.
dry ether
RMgX + H2O —————> R-H + Mg(OH)X
alkyl magnesium halide (alkane) (Hydroxy magnesium halide)
- From salts of Carboxylic acid
- By Kolbe’s electrolytic method
Electrolysis of aqueous conc. solution of sodium or potassium salt of carboxylic acid gives alkanes.
>RCOONa—-Sodium salt
>RCOOK—–Potassium salt
RCOONa——–> RCOO– + Na+
anion cation
During electrolysis;
Electrode reaction occurs as
At anode:
RCOO– – e ——–> RCOO ———> R-R + 2CO2
unstable alkane
At cathode:
Na+ + OH– ———> NaOH
- By Heating Na-salt of Carboxylic acid
When Na-salt of carboxylic acid is heated with soda lime (NaOH & CaO), alkane is obtained having one carbon less than salt by removal of a molecule of CO2. This reaction is also known as decarboxylation.
RCOONa + NaOH R-H + Na2CO3
Eg:
Sodium ethanoate (methane)
This is the principle reaction for laboratory preparation of methane gas.
- From Metal Carbides
Metal carbide like aluminium carbide (Al4C3) and beryllium carbide (Be2C) reacts with pure water to produce methane.
Al4C3 + 12H2O ———-> 4Al(OH)3 + 3CH4
USES AND TEST FOR ALKANES
Alkanes are unreactive and are mainly used as fuels.
Alkenes are much more reactive than alkanes
because alkenes are unsaturated
(they have a reactive double bond).
Alkenes are the starting material for many different chemicals.
They are extremely useful to the chemical industry.
Ethene and propene are the starting materials for polymers,
giving poly(ethene) and poly(propene) – see polymers.
How to Tell an Alkane from an Alkene?
An alkene may be distinguished from an alkane by
shaking the hydrocarbon with bromine water.
Bromine water is brown or orange, and will lose its colour
when it reacts with the double bond of an alkene.
Bromine water will stay brown with an alkane
because an alkane has no double bonds.
Bromine adds across the double bond of an alkene
to form a colourless dibromo alkane.
This is an example of an addition reaction.
What is an Addition Reaction?
An addition reaction occurs when two or more reactants
join together to form a single product.
Other examples of addition reactions are
the hydrogenation of vegetable oils,
addition polymerisation and the hydration of ethane
REVISION EXERCISES
- Alkanes does not undergo addition reaction because ———-
- Give the name and formula of two gaseous alkanes and two liquid alkanes.
3.What is the major use of the alkanes?
4.Describe the chemical properties of Alkanes.
WEEK 8
ALKENES
Alkenes
Alkenes are a homologous series of hydrocarbons that contain a carbon-carbon double bond. The number of hydrogen atoms in an alkene is double the number of carbon atoms, so they have the general formula .
For example, the molecular formula of ethene is , while for propene it is .
Here are the names and structures of four alkenes:
Alkenes are unsaturated, meaning they contain a double bond. This bond is why the alkenes are more reactive than the alkanes.
General Methods of Preparation of alkenes
Alkenes are usually prepared from either alcohols or haloalkanes (alkyl halides).
Dehydration of Alcohols
Alkenes are obtained by the dehydration of alcohols. The dehydration of alcohols can be affected by two common methods.
1.By passing the vapors of an alcohol over heated alumina.
2.By heating an alcohol with concentrated mineral acid, such as concentrated H2SO4 or concentrated H3PO4. Anhydrous zinc chloride can also be used as a dehydrating agent.
By passing the vapors of an alcohol over alumina (Al2O3) at 623 K (350°C).
The order of the ease of dehydration of alcohols is: tertiary > secondary > primary. Secondary and tertiary alcohols are best dehydrated by dilute sulfuric acid.
By heating an alcohol with concentrated sulfuric acid at 453 K (180°C).
Other dehydrating agents like phosphoric acid and anhydrous zinc chloride may also be used.
Example
Cyclohexanol on dehydration gives cyclohexene.
cyclohexanol cyclohexene
The loss of water from an alcohol to give an alkene does not occur in just one step; a series of steps are involved in the mechanism of dehydration of alcohols. In the dehydration reaction given above, the following steps are involved.
1.First, the acid protonates (adding a proton or H+) the alcohol on the most electronegative atom, namely oxygen. This process is usually reversible.
2.In the second step, the protonated alcohol loses water to give a positively charged species known as a carbonium ion or carbocation.
3.Finally the carbonium ion loses a proton to give alkene.
The mechanism of dehydration of ethyl alcohol is described below.
From haloalkanes (or, alkyl halides)
Alkenes can be obtained from haloalkanes (alkyl halides). These haloalkanes are usually bromo and iodo and less commonly, chloro derivatives.
By dehydrohalogenation of haloalkanes
Haloalkanes on heating with alcoholic potash loses one molecule of hydrogen halide to give alkene.
Example
Example
If two alkenes may be formed due to dehydrohalogenation of a haloalkane, the one which is most substituted is the main product.
Example
For example, dehydrohalogenation of 2-bromobutane gives,
The order of reactivity of haloalkanes in dehydrohalogenation is, Tertiary > Secondary > Primary. Note: Reactions in which a small molecule like H2O or HX is lost are known as elimination reactions.
From vicinal dihaloalkanes
Vicinal dihaloalkanes are those dihalogen derivatives of alkanes in which two halogen atoms are on the adjacent carbon atoms. Alkenes can be obtained from vicinal dihaloalkanes by dehalogenation. When such a dihaloalkane is heated with zinc in methanol, an alkene is formed.
Example
1,2-dibromoethane gives 1,2-dibromoethane ethene
Reduction of Carboxylates: Kolbe’s Electrolytic method
Sodium or potassium salt of a dicarboxylic acid on electrolysis gives an alkene. an alkene is generated when an aqueous solution of sodium or potassium salt of a dibasic acid (with adjacent carboyxlic groups) is electrolyzed.
Example: Electrolysis of Sodium Succinate
The electrolysis of sodium succinate gives ethene.
Oxidation at the anode
Reduction at Cathode
2H2O+2e−→2OH−+H2(g)
Hydrogenation of Alkynes
Alkenes can be easily obtained by hydrogenation of alkynes. An alkyne on controlled hydrogenation with hydrogen in the presence of Ni or Pd at 200°C give a corresponding alkene.
CnH2n−2+H2−→−−−−Ni,200CCnH2n
Example
Ethyne gives ethene on hydrogenation.
Testing for alkenes
The presence of the C=C double bond allows alkenes to react in ways that alkanes cannot. This allows us to tell alkenes apart from alkanes using a simple chemical test.
Bromine water is an orange solution of bromine. It becomes colourless when it is shaken with an alkene. Alkenes can decolourise bromine water, but alkanes cannot. The slideshow shows this process.
The reaction between bromine and alkenes is an example of a type of reaction called an addition reaction. The bromine is decolourised because a colourless dibromo compound forms. For example:
ethene + bromine → dibromoethane
C2H4 + Br2 → C2H4Br2
Other addition reactions of alkenes:
- Hydrogen can be added to a C=C double bond. This has the effect of ‘saturating’ the molecule, and will turn an alkene into an alkane. For example: C2H4 + H2 → C2H6
- If steam (H2O) is added to an alkene, an alcohol is made. For example: C2H4 + H2O → C2H5OH
Chemical properties of alkene
Alkenes are more reactive than alkanes due to the presence of a double bond. The carbon-carbon double bond consists of a strong bond and a weak p bond. The typical reactions of alkenes involve the breaking of this weaker p bond, viz., and formation of two sigma (s) bonds.
Such reactions are called addition reactions and are initiated by an electrophile, proceeding through ionic mechanism. However, some addition reactions proceed through free-radical mechanism.
Higher alkenes contain a long chain of carbon. That part of the chain that forms an alkane-like structure (consisting of C-C bonds), may undergo substitution reaction as also shown by alkanes. Some characteristic reactions shown by alkenes are described below:
Combustion
Alkenes, like alkanes, are highly combustible. Alkenes burn with a luminous flame to give carbon dioxide and water. The flame becomes luminous because of the higher carbon content of alkenes than alkanes. Their combustion reactions are exothermic.
Due to the luminosity of the flame, the lower alkenes may be used as illuminants.
Addition reactions
The p electrons of the carbon-carbon-double bond are available to an electrophile (any species seeking electrons). Thus, the addition reactions shown by alkenes are in fact electrophilic addition reactions.
addition product
Some addition reactions proceed through free-radical mechanism.
Addition of hydrogen
Alkenes add hydrogen in the presence of platinum or nickel catalyst, to form alkanes. The reaction termed as hydrogenation, is an exothermic reaction.
CnH2n+ H2 CnH2n+2 + heat
This is known as Sabatier-Senderens reduction.
CH2=CH2 + H2 CH3-CH3 + 132.2 kJ
ethene ethane
Addition of halogens
Alkenes react with halogens to form dihaloalkanes. The order of reactivity is, chlorine > bromine > iodine. Simply mixing together the two reactants, usually in an inert solvent like carbon tetrachloride, best carries out the reaction.
Alkene dihaloalkane
ethene 1,2-dibromoethane
propene 1,2-dibromopropane
Addition of bromine is useful for the detection of the carbon-carbon double bond. When a 5% solution of bromine in carbon tetrachloride is added to an alkene, it gets decolorized. This indicates the presence of a double bond in the molecule. This test is called ‘bromine test’.
Mechanism of halogen addition
The addition of halogen across the double bond takes place through the following steps. Taking an example of ethene,
- Ethene molecule undergoes electromeric effect
- Due to its close proximity to the carbon-carbon double bond, the non-polar halogen molecule gets polarized
- The polarized halogen molecule forms a transition-state complex with ethene molecule.
- The X- ion attaches itself to the positively charged carbon.
Addition of halogen acids
Alkenes with concentrated aqueous solution of halogen acids give haloalkanes. The order of reactivity is,HI > HBr > HCl
For example:
Ethene gives
Ethane haloethane
2-butene with HBr gives
2-Butene 2-bromobutane
Thus, symmetrical alkenes give only one product, due to the equivalence of the two carbon atoms (the H and X may add to the molecule in any way).
In asymmetrical alkenes, the addition of a halogen acid takes place in a manner where by the halogen atom (the negative part of the molecule to be added) adds to the carbon atom, which has lesser number of hydrogen atoms on it. For example, in the case of propene, the product obtained is 2-iodopropane and not 1-iodopropane.
The I being negative part of the added molecule, goes to the carbon number 2 because it has only one H-atom on it. (lesser number of H-atom)
This rule of the addition of halogen acids to an asymmetrical alkene is known as Markownikoff’s rule (1869).
Markownikoff’s rule
This is an empirical rule but it may be explained theoretically on the basis that the addition occurs by a polar mechanism. For example, the addition of HI to propylene. Since a methyl group is electron-repelling, the propylene molecule is polarized as follows.
2-iodopropane
Hence, the proton of the hydroiodic acid gets attached to the negatively charged carbon and the iodide ion to the positive carbon.
Peroxide effect
The mode of addition of hydrogen bromide to unsymmetrical alkenes in the presence of oxygen and peroxides is contrary to Markownikoff rule. This addition of HBr to unsymmetrical alkenes against the Markownikoff’s rule is known as peroxide effect, or anti-Markownikoff’s rule.
For instance, the reaction of propene with HBr in the presence of peroxides, forms 1-bromopropane instead of 2-brompropane.
CH3-CH=CH2 + HBrCH3-CH2-CH2Br1 -bromopropane
The mode of addition of hydrogen chloride or hydrogen iodide is not affected by the presence of peroxides.
Addition of sulphuric acid
In accordance to Markownikoff’s rule alkenes readily add concentrated sulphuric acid to form alkyl hydrogen sulphates. For example,
Ethene gives,
ethane sulphuric acid ethyl hydrogen sulphate
Propene gives,
Iso-propyl hydrogen sulphate
An alkyl hydrogen sulphate on boiling with water gives the alcohol and sulphuric acid. Alcohols are prepared from alkenes obtained from the cracking of petroleum. For example,
ethyl hydrogen sulphate ethanol
Addition of hypohalous acids
Hypohalous acid (HOX) in accordance with the Markownikoff’s rule, add to the molecule of an alkene at the double bond. For example,
ethene hypochlorous acid 2-chloroethanol(ethylene chlorohydrin)
propene 1-chloro-2-propanol(propylene chlorohydrin)
Addition of water (Hydration of alkenes)
Water molecule adds to an alkene molecule across the double bond in the presence of dilute acids and a catalyst. For example, ethane gives ethanol when a mixture of ethene and steam is passed over phosphoric acid and silica under a pressure 65 atm, and at 300C.
ethene ethanol
Addition of oxygen
Lower alkenes are mixed with air and passed under pressure over a silver catalyst at 200-400°C. This gives epoxides by adding one atom of oxygen across the double bond. The epoxides so obtained are used in detergents.
ethene ethene epoxide
Addition of ozone
Ozonides are formed when alkenes add a molecule of ozone across the double bond. For example, ethene gives ethene ozonide.
ethene ethene ozonide
Ozonides on hydrolysis with water in the presence of a reducing agent give aldehydes.
The oxidation of alkenes by ozone followed by decomposition of the formed ozonide with water, is termed as ‘ozonolysis’. The nature of the products (aldehydes and ketones) formed due to ozonolysis depends upon the location of the double bond in the parent alkene. Therefore, this reaction provides a very convenient way of locating the position of the double bond in any molecule.
As in the above example, the only product formed upon the hydrolysis of ethene ozonide is formaldehyde (containing one carbon unit each) hence the double bond has only one carbon unit on either side.
In the following example,
The products of ozonolysis are, acetone (3 carbon unit) and acetaldehyde (2 carbon unit).
It implies the location of the double bond being between two carbon chains of 2 carbon and 3 carbon atoms.
Oxidation
Alkenes can be readily oxidized, but the nature of the products depends upon the oxidizing agent used.
4″>
With cold, alkaline KMnO4
When alkenes are oxidised with cold, alkaline KMnO4, dihydroxy compounds (diols or glycols) are formed. The KMnO4 gets decolorized. This reaction is therefore, used as Bayer’s test for unsaturation (the presence of double or triple bonds) in any molecule.
Ethene gives ethane-1,2-diol.
ethane ethane-1,2-diol
4-Or-K2Cr2O7″>
With acidified KMnO4 Or K2Cr2O7
Acidified potassium permanganate (or potassium dichromate) oxidises the dihydroxy compound so produced in reaction to ketone and/or carboxylic acid. For example,
Substitution reactions
At elevated temperatures (500°C), higher alkenes give substitution products with chlorine. For example,
CH3-CH=CH2 + Cl2 ClCH2-CH=CH2 +HCl
propene 3-chloropropene
Branched-chain alkenes give substitution reaction easily. For example isobutene gives substitution product with chlorine even at room temperature.
Polymerization
Addition polymerization is a process by which a large number of molecules of the same species join together (without the elimination of simple molecules like HX, H2O, etc.,) to form a giant molecule, called a polymer. Alkenes undergo addition polymerization when heated under pressure, in the presence of suitable catalysts. When ethene is heated to 1000C under 1000 atm pressure in presence of oxygen, we get polyethene
Similarly, when vinyl chloride is polymerized in the presence of peroxide catalyst, it forms polyvinyl chloride (PVC)
Isomerization
Alkenes when heated alone at high temperatures (500-700°C) or at lower temperatures (200-300°C) isomerizes in the presence of catalyst, such as Al2(SO4)3. Alkenes isomerism due to,
- The shifting of the double bond which tends to move towards the center of chain, e.g., pentene-1 isomerizes to pentene-2.
CH3-CH2-CH2-CH=CH2CH3-CH2-CH=CH-CH3
pentene-1 pentene-2
- The migration of a methyl group, e.g., butene-1 isomerizes to 2-methylpropene (iso-butene).
When alkenes are oxidised with cold, alkaline KMnO4, dihydroxy compounds (diols or glycols) are formed. The KMnO4 gets decolorized. This reaction is therefore, used as Bayer’s test for unsaturation (the presence of double or triple bonds) in any molecule.
Ethene gives ethane-1,2-diol.
ethane ethane-1,2-diol
Polymerisation of alkenes
The monomer molecules add together to make polymer molecules. Nothing in the monomer molecule is left out. Addition polymers are made from monomers that have a double bond between carbon atoms. These monomer molecules are generally alkenes such as ethene.
Styrene (monomer)
Polystyrene
Uses
- In the manufacture of ethyl alcohol and ethylene glycol which are used as important starting materials in the manufacture of synthetic fibres.
- Manufacture of plastics like polythene.
- In artificial ripening of fruits like bananas, apples and oranges.
REVISION EXERCISES
- The gas ethane C2H4 is obtained by the CRACKING of oils and natural gas. What do you understand by cracking?
- Give three properties of ethane (chemical and physical).
- Write the structural formulae of ethane.
4The volume of ethylene at S.T.P produced from 3.2g of calcium carbide.(Ca=40, O=16, C=12,H=1, Molar volume at S.T.P.=22.4dm3).Calculate the mass of water that would be required to produce 0.5 mole of ethylene.
WEEK 9
ALKYNES
INTRODUCTION
GENERAL MOLECULAR FORMULA CnH2n-2
Molecular formula = C2H2
Empirical formula = CH
Molecular mass = 26
Empirical mass = 13
Common name = Acetylene
Homologous series = Alkynes
STRUCTURE OF ETHYNE
COMPOSITION OF ETHYNE MOLECULE:
Ethyne molecule consists of two C-atoms and two H-atoms (C2H2).
BOND LENGTH:
The C–H bond is 1.2Ao.
BOND ANGLE:
HCC bond angle is 180o.
METHODS OF PREPARATION
BY THE HYDROLYSIS OF CARBON CARBIDE:
CaC2 + 2H2O C2H2 + Ca(OH)2
BY TETRA CHLOROETHANE:
Cl2CH-CHCl2 + 2Zn C2H2 + 2ZnCl2
CHEMICAL PROPERTIES
COMBUSTION REACTION:
Ethyne burns in air with a luminous smoky flame and hence used for lightening purpose.
2C2H2 + 5O2 4CO2 + 2H2O + heat
ADDITION REACTIONS:
Addition of Hydrogen:
C2H2 CH2=CH2 C2H6
Addition of Chlorine:
C2H2 +Cl2 Cl-CH=CH-Cl Cl2CH-CHCl2
Addition of Hydrogen Halide:
C2H2 + HBr CH2=CH-Br + HBr CH3-CHBr2
PHYSICAL PROPERTIES
Ethyne is a colourless gas with a characteristic smell.
It has a melting point -81oC.
It has a boiling point -84oC.
It is significantly soluble in water. However readily soluble in organic solvents.
USES OF ETHYNE
It is used in welding to produce oxy-acetylene flame of temperature about 3000oC.
It is used in the synthesis of useful compounds such as ethanol, ethanoic acid, PVC, acetaldehyde
Physical properties of alkynes
Physical state
The first three members (ethyne, propyne and butyne) are colourless and odorless gases. Due to the presence of phosphine as an impurity ethyne (acetylene) has garlic smell. The next eight members are liquids, and higher members are solids under normal conditions of temperature and pressure.
Solubility
Alkynes are insoluble in water, but are fairly soluble in organic solvents such as, alcohol, ether, acetone etc.
Melting and boiling points
The melting and boiling points of alkynes increase with molecular mass. Melting boiling points of some alkynes are,
Ethyne/acetylene (CH º CH), – 83oC or 190 K
Propyne (CH3-CºCH), – 27oC or 246 K
1 – Butane (CH3-CH2-CºCH), 8oC or 281 K
2 – Butane (CH3-CºC-CH3), 29oC or 302 K
1 – Pentyne (CH3-CH2-CH2-CºCH) 48oC or 321 K
2 – Pentyne (CH3-CºC-CH2-CH3) 55oC or 328 K
Laboratory preparation of ethyne
Ethyne is prepared by the action of water on calcium carbide.
Preparation of ethyne
The gas thus produced is collected by the downward displacement of water.
General method of preparation of alkynes
1.Ethyne is made industrially from hydrocarbons obtained by cracking petroleum. The thermal cracking of butane with steam as a diluent. The first reaction is
3 C4H10 + 2 H2O ==> 2 HCCH + 2 C2H4 + 2 CH4 + 2 CO + 7 H2 Ethyne
Subsequent reactions in this gas stream are
C2H4 ==> HCCH + H2
and
2 CH4 ==> HCCH + 3H2
2,Ethyne is also made industrially by the combustion of natural gas, which is mainly methane using insufficient oxygen. The heat evolved in the incomplete combustion is used to heat a further supply of methane to about 1773 degK.
2 CH4 + O2 ==> 2 CO + 2 H2
The reaction time is very short (between 0.01 and 0.05 seconds). The optimum yield of ethyne is obtained by rapid cycling of the products.
3.Ethyne is also made industrially by the arc process in which a temperature of 1773 degK is achieved by an electric discharge. The feedstock is usually a mixture of C3 hydrocarbons and C4 hydrocarbons and the products are mainly ethyne, methane and hydrogen. Contact time is again very short.
The initial step in the purification of ethyne from these thermal processes to absorb it selectively into a suitable solvent, and then to separate the ethyne by fractional distillation. The solvent for the extraction is recycled.
Properties of Alkynes.
A Physical properties.
Alkynes, having a short bond, end up carrying polarized bonds/atoms attached to either side of this functionality. The proximity of the supposedly remote carbon nucleus is taken to be the main cause of such polarizations. In addition to this, the electron cloud, resulting from the four pi and the two sigma electrons, is very firmly attracted to the closely positioned carbon atoms. There will hence be some degree of reluctance for these electrons to participate in electrophilic additions. Where an H atom is directly attached to the triple bond i.e. in the so-called terminal alkynes, this atom is very easily lost as its bonding electrons are already drawn in towards the triple bond. If this were another carbon atom, then this atom would be somewhat deficient in electron density for the same reason. It is therefore not unreasonable to assume that this will account for the alkynes generally having a higher melting/boiling point than their alkene counterparts as polarizations within the molecules give rise to stronger intermolecular dipole-dipole interactions.
By way of a general comparison of some of the properties of the hydrocarbons, with particular emphasis on some selected atoms directly attached to the functionalities, the following table has been drawn.
B Chemical Properties.
The chemistry of alkynes depends on the physical factors briefly outlined in the above section. One would think that with so much electron density about, alkynes would be more willing to participate in electrophilic additions. This is however not what one observes although addition reactions are seen to take place (but, one might add, not with the vigour initially expected!) The high coulombic attractions (i.e. the attractions between oppositely charged species), between the carbon nuclei, in very close proximity, and the surrounding electronic charge accounts for the reluctance of these electrons to ‘get involved’. Thus bromine water will be decolorized by alkynes but this is in no way immediate, as it is for alkenes – and this despite there being two pi bonds available for reaction!
Also, as has already been mentioned, terminal alkynes have acidic hydrogen atoms which are easily replaced by metal ions.
- Combustion
Alkynes have a stronger carbon to carbon bond as their functionality and this generally results in incomplete combustion when these compounds are burnt in air. Their flames tend to be very sooty as though either the temperature is not high enough, or the flame is not oxidizing enough to give efficient conversion of the alkynes to CO2 and H2O, or both. Very sooty luminous flames are characteristic of compounds having a high degree of unsaturation e.g. in burning alkynes. However, if the alkyne is burnt in pure oxygen a very high temperature can be reached and an important example of this is the use of ethyne in ‘oxy-acetylene’ burners used for welding metals.
- Addition Reactions
(a) Hydrogenation.
The reduction or hydrogenation of alkynes can be carried out in either of two ways:
- Catalytic : where a hydrogen molecule initially adds to the alkyne in a cis fashion to give the alkene which immediately reacts further giving the saturated compound, the alkane. This reaction, as has already been stated, requires the use of a catalyst such as Raney nickel.
or
- Chemical : If the hydrogen is that which is generated ‘in situ’ by the reaction of sodium with ammonia, this hydrogen adds to the triple bond in a trans fashion and the reaction does not proceed to the alkane under these conditions.
(b) Halogenation.
Reactions of the alkynes with halogens exactly follows the pattern seen for the alkenes. In these addition reactions with the halogens the vigour for the reaction is F2>Cl2>Br2>>I2. Chlorine reacts with ethyne explosively giving the 1,1,2,2-tetrachloroethane.
Bromine participates in an analogous reaction but the product depends on the state of the bromine used. With pure bromine liquid, the same reaction as for the chlorine is seen while an aqueous solution of bromine only gives the 1,2-dibromoalkene in a slow reaction (unlike the alkenes). [Reaction with iodine reaction is difficult]
(c) Hydrohalogenation – Addition of Hydrogen halide (HI>HBr>HCl>>HF).
The addition of hydrogen halide to the alkyne takes place in two steps in sequence and it is sometimes possible to isolate the product after only the first addition. For example, reaction of ethyne with HCl using Hg2Cl2 as catalyst gives an important monomer, chloroethane, for use in formation of the thermoplastic poly(chloroethane) [or PVC as it was previously known]. The second addition then gives the 1,2-dichloroethane (as this reaction then obeys Markovnikov`s rule).
(d) Hydration – Addition of H2O.
This addition reaction (also governed by the Markovnikov rule where applicable) uses mercury(II) sulphate together with dilute sulphuric acid as the catalysts and the reaction is easily carried out at 60OC. The product of addition of water (1 mole) is an unstable ‘enol’ which immediately reverts to its ‘keto’ structure which is preferred.
(e) Oxidation
Alkynes such as ethyne burn with a very sooty flame and this feature is used as part evidence for the high degree of unsaturation. (Ethyne gives a flame with 92%C but in pure oxygen it is capable of giving efficient combustion raising the temperature of the flame to a whopping 2000OC – capable of melting metals!) Synthetically useful oxidation reactions are however few and are limited to the following:
Oxidation using potassium permanganate KMnO4
As was seen for the reactions of alkenes with KMnO4, this reaction is very sensitive to conditions used. Care will have to be taken if acidic MnO4- is used as the product can be oxidized further.
For other alkynes, the triple bond breaks to give carboxylic acids :
Despite its unsaturation, ethyne decolorizes acidified permanganate only slowly.
(f) Polymerisation
Though ethyne and similarly other alkynes can theoretically produce polymers with a high molecular weight, only short chains are obtained. With ethyne for instance, the trimer forms readily as the alkyne is passed through a hot tube. This trimer is the very stable aromatic compound benzene :
(g) Formation of Alkynides (Acetylides)
As has been discussed above, terminal alkynes readily replace the end-hydrogen with a metal ion. This feature of the terminal alkynes has been used for a number of important reasons such as preparation of synthetic intermediates, purification of alkynes and analytical confirmation of the presence of a terminal triple bond.
- i) When ethyne is passed over heated sodium only one of the terminal hydrogens is replaced initially. Heating this monosodium salt will give the disodium salt and ethyne.
Na2C2 reacts violently with water to release ethyne and also forms sodium hydroxide.
- ii) Ethyne similarly reacts with a ammoniacal solutions of copper(I) chloride and silver chloride to form characteristically coloured precipitates. These reactions are used as tests for terminal alkynes
The explosive-when-dry copper and silver ethynide precipitates can be made to release the pure ethyne by addition of acid such as dil. HCl (-other acids may react explosively!) This reaction can easily be used to purify / separate alkynes from a mixture.
Tests for alkynes.
As we`ve seen before, elemental analysis only gives presence of carbon and hydrogen if a simple alkyne is analysed. Empirical formulae calculations already indicate the presence of the unsaturation.
Chemical tests for the triple bond functionality must then be performed and these include the following:
(a) A luminous, very sooty flame is given if alkynes are present.
(b) Reaction with bromine water and with acidified potassium permanganate both decolorize very slowly (unlike the alkenes)
(c) If terminal alkynes are being examined, precipitates are obtained with ammoniacal silver nitrate (pale yellow-light brown ppt) and with ammoniacal copper(I) chloride solution (which gives an orange/red ppt)
(Note If the triple bond is not at the end of the chain, its confirmation is somewhat more involved)
BENZENE
Aromatic compounds
These carbocylic compounds contain at east one benzene ring i.e., a ring of six carbons atoms with alternate double and single bonds. Aromatic compounds are called so because many of them possess a fragrant smell.
Typical examples of aromatic compounds are given below:
Benzene
The aromatic compounds may have a side-chain or a functional group attached directly to the ring. For example,
Toluene Phenol Nitrobenzene Benzaldehyde
The aromatic compounds may also contain more than one benzene rings fused together
Naphthalene Anthracene
There are two classifications of aromatic compounds.
Nuclear Substituted Compounds
When the functional group or any substituent, in aromatic compounds is directly attached to the benzene ring, it is a called nuclear substituted compound. Such compounds are named as the derivatives of benzene under the IUPAC system. However, the common names of many such compounds are retained by IUPAC.
Sidechain Substituted Compounds
Aromatic compounds where the functional group is present in the sidechain of the ring are called sidechain substituted compounds. Sidechain substituted compounds are named as the phenyl derivatives of the corresponding aliphatic compounds.
Naming Benzene Derivatives
Only one kind of monosubstituted derivatives are possible in benzene rings as all six hydrogen atoms are equivalent. For example, there is only one toluene. It does not matter where the methyl group is attached because all the following arrangements are equivalent.
When two same or different monovalent substituents, are present on a benzene ring, the following three arrangements are possible.
For the same substituent (A)
ortho (or, 1,2-) meta-(or, 1,3-) para- (or, 1,4)
For different substituents (A and B)
ortho (or, 1,2-) meta-(or, 1,3-) para- (or, 1,4)
These arrangements are named as follows:
The compound containing the two groups on the adjacent sites is called ‘ortho’; it is denoted as ‘o-‘. In the IUPAC system, the ortho position is designated as 1,2-.
The compound containing the two groups on alternate sites is called ‘meta’: it is denoted as ‘m-‘. In the IUPAC system,the meta position is designated as 1,3-.
The compound containing the two groups diagonally opposite to each other is called ‘para’: denoted as ‘p-‘. In the IUPAC system, the para position is designated as 1,4-.
For example, the three xylenes are named as,
o-xylene m-xylene p- xylene
(1,2-dimethylbenzene) (1,3-dimethylbenzene) (1,3-dimethylbenzene)
In the case of trisubstituted derivatives, the nature of the substituted groups determine the number of arrangements. When the three substituent groups are identical (say, A), three arrangements are possible. These are termed as follows.
vicinal (vic-) unsymmetrical (unsym-) symmetrical (sym-)
For a trisubstituted product, if the two substituents are identical and the third different, then six products are possible. When all the three groups are different, ten products are possible. Since, naming each individual compound is not possible, it was found convenient to indicate the position of any substituent by the numeral indicating the serial number of the carbon atom in the ring, to which that substituent is attached.
Numbering the Carbon Atoms in the Ring
The numbering of carbon atoms in the ring (or nucleus) is done as follows.
- When there is only one substituent on the ring, there is only one compound possible. Thus, numbering of the carbon atoms of the nucleus does not arises.
- If there are two or more substituents, then numbering is in the alphabetical order of the substituents on the carbon atoms. The prefixes such as ‘di’, ‘tri’, ‘cyclo’, ‘iso’, etc., are ignored while arranging the substituents alphabetically.
- When two or more functional groups are present, then the principal functional group is assigned the number 1. The order of priority of the functional groups is the same as done for aliphatic polyfunctional compounds.
For the sake of convenience, the ring is oriented in such a way that position 1 is at the top and numbering is done in a clockwise or anticlockwise manner whichever gives lower numbers to the other substituents.
This is illustrated through the following example. The IUPAC names are written in bold letters.
(o-xylene) (m-xylene) (p-xylene)
1,2-dimethylbenzene 1,3-dimethylbenzene 1,4-dimethylbenzene
Names of some typical aromatic compounds are given below:
Aromatic hydrocarbons (arenes)
toluene o-xylene m-xylene p-xylene
Methylbenzene 1,2-dimethylbenzene 1,3-dimethylbenzene 1,4-dimethylbenzene
1,3,5-trimethylbenzene 2-phenylpropane(cumene) phenylethene (styrene)
Anthracene Phenonthrene
Halogen derivatives
2-chlorotoulene 1,2-dichlorobenzene phenyl chloromethane
Hydroxy derivatives:
Phenols and aromatic alcohols
2-methyl phenol phenylmethanol 1,4-dihydroxy benzene 2,4,6-trinitrophenol(o-cresol) (picric acid)
Aldehydes and Ketones
Nuclear substituted
Benzaldehyde Methyl phenyl ketone Diphenyl ketone(Acetophenone) (Benzophenone)
Carboxylic acids
Nuclear substituted
benzoic acid 2-methylbenzoic acid 2-hydroxybenzoic acid 1,4-benzenedicarboxylic acid(o-toluic acid) (o-salicylic acid) (terephthalic acid)
Acid derivatives
Benzoyl chloride Benzamide Phenyl benzoate
Benzoic anhydride Ethyl-4-bromobenzoate
Alkoxy derivatives
Methoxy benzene 1-methoxy- phenoxybenzene4-nitrobenzene
Amines
Aminobenzene 2-Amino toluene Benzyl amine
Nitro derivatives
nitrobenzene 1,3-dinitrobenzene 2,4,6-trinitrotoluene(m-nitrobenzene)
Nitriles and Carbylamines
Benzonitrile Phenylcarbylamine(phenyl isocyanide) (phenyl cyanide)
Sulphonic acids
benzenesulphonic acid benzene-1,3-disulphonic acid
Aromaticity
Aromatic compounds are those, which resemble benzene in chemical behavior. These compounds contain alternate double and single bonds in a cyclic structure. They undergo substitution reactions rather than addition reactions. This characteristic behavior is called aromatic character or aromaticity the criteria for which are as follows:
- Contains a cyclic cloud of delocalized p electrons above and below the plane of the molecule.
- Electrons cloud must contain a total of (4n+2) a electrons, where p is an integer equal to 0,1,2,3 ………..
This is known as ‘Huckel rule’ according to which the aromatic compounds have delocalized electron cloud of p electrons of 2 or 6 or 10 or 14 electrons.
For example, benzene (6p electrons), naphthalene (10p electrons) and anthracene (14p electrons) are aromatic compounds.
Benzene Naphthalene Anthracene
6p electrons 10p electrons 14p electrons
Similarly, cyclopentadienyl anion and cycloheptatrienyl cation (tropylium ion) are also aromatic because these contain 6p electrons.
Cyclopentadienyl anion Cycloheptatrienyl cation
6p electrons (Tropylium ion) 6p electrons
Heterocyclic compounds such as pyrrole, furan, thiophene and pyridine also behave as aromatic because all have 6p electrons.
Furan Thiophene Pyrrole Pyridine
Structure of benzene
The molecular formula of benzene has been found from analytical data, to be C6H6. Relatively higher proportion of carbon and addition of chlorine to benzene molecule indicate it to be an unsaturated compound. Depending on the various facts available to scientists from time to time, many structures for benzene had been proposed. Some are described below.
Open Chain Structure
Based upon observable facts given above and the tetravalency of carbon, the following open chain structures were proposed for benzene.
Drawbacks of open chain structure
The open chain structure for benzene was rejected due to the following reasons:
- Addition reactions usually given by alkenes and alkynes are not given by benzene.
- Benzene forms only one kind of mono- substituted product. An open chain structure however, can form more than one kind of monosubstituted product as shown below:
- The open chain compounds do, not give reactions such as Friedel-Craft reaction, nitration, sulphonation.
- On reduction with hydrogen in the presence of Ni at 200°C, actually a cyclic compound cyclohexane is obtained.
These facts suggest a ring structure for benzene.
Ring structure of benzene
After taking into account account the above observed facts, Kekule (1865) suggested a ring structure for benzene. According to him, six carbon atoms occupied six corners of a regular hexagon in benzene and each carbon carried one hydrogen atom. To satisfy the tetravalency of carbon, the system consisted of alternate single and double bonds. Kekule’s formula is shown below.
Defects in Kekule’s formula
While Kekule’s formula explained most of theory served facts for benzene, it could still not explain the following facts,
- The saturated nature of benzene.
- In actuality only one kind of ortho derivatives are known, but according to Kekule’s formula, there can be two ortho positions.
The defect of having two ortho positions was explained by proposing that the positions of the double bonds in benzene are not fixed. Instead, the double bonds in the benzene molecule keep changing their positions and thus all positions in benzene molecule become identical.
Chemists generally used the Kekule’s structure as late as 1945. Many ring structures for benzene have been proposed after Kekule’s structure. Some of them are,
Claus diagonal Dewar’sformula(1867) formula(1867)
Resonance hybrid structure of benzene
The currently accepted structure was developed by the application of the theory of resonance proposed in 1933. This theory states that benzene is a resonance hybrid of the following canonical forms.
Since, the forms I and II are the most contributing, hence benzene is represented as a hybrid structure of these two structures, i.e.,
Resonance hybrid
Evidences which support resonance structure of benzene
The following facts support the resonance structure of benzene:
- The carbon-carbon bond length in benzene is identical at 139 pm, for all bonds. This value is intermediate between the bond lengths for C-C bond (154 pm) and C = C (134 pm).
- A regular hexagonal structure for benzene is obtained by X-ray diffraction, which gives a C-C bond length of 139 pm.
Large resonance energy
Due to resonance, the p-electron charge in benzene gets distributed over greater area, i.e., gets delocalized. Delocalization results in the energy of the resonance hybrid decreasing relative to the contributing structures, by about 150 kJ mol-1. This decrease in energy is called resonance energy. The unusual stability of benzene is due to this resonance stabilization.
Preparation of Benzene
Benzene and its homologues may be prepared by the following methods.
By the decarboxylation of sodium benzoate
This method is used in the laboratory to obtain benzene. Sodium benzoate is heated with soda-lime and when it gets decarboxylated (removal of carbon dioxide) benzene is obtained.
sodium benzoate sodalime Benzene
By heating phenol with zinc
When phenol vapours are passed over heated zinc dust, benzene is formed.
phenol benzene
By the polymerization of ethyne (acetylene)
When ethyne (acetylene) is passed through a red hot copper tube, it polymerises to benzene.
By the reduction of benzene diazonium chloride
Benzene is formed on the reduction of benzene diazonium chloride with sodium stannite or hypophosphorus acid.
C6H5NCl- + H3PO2 + H2OC6H6 + N2 + H3PO3 + HClbenzenediazoniumchloride
By the hydrolysis of sulphonic acid
Benzene sulphonic acid on hydrolysis with superheated steam gives benzene.
C6H5.SO3H + H2O C6H6 + H2SO4
benzene sulphonic acid benzene
Physical properties of benzene
- Benzene is a colorless liquid (melting point 5.5°C, boiling point 80.4°C) with a characteristic smell of petrol.
- It is immiscible with water, but dissolves in organic solvents.
- It is lighter than water: density 0.87 g cm-3
- Its vapors are toxic.
Addition reactions of benzene
Benzene gives some addition reactions. Some typical reactions are:
Addition of hydrogen
Benzene on reduction with hydrogen under pressure in the presence of finely divided nickel at 200°C, gives an addition product hexahydrobenzene (cyclohexane).
cyclohexane
Addition of chlorine
Chlorine adds on to benzene at its boiling point, in the presence of bright sunlight, to give hexachloride.
benzene hexachloride
Addition of ozone
Benzene reacts slowly with ozone to form triozonide. Triozonide on hydrolysis with water gives glyoxal.
Pyrolysis
Benzene when passed through a red hot iron tube gives diphenyl.
diphenyl
Electrophilic substitution reactions of benzene
Benzene gives substitution reactions as described below.
Nitration
When benzene is heated with concentrated HNO3 in presence of concentrated sulphuric acid at 333 K, we get nitrobenzene.
[A mixture of conc. HNO3+conc.H2SO4 is called as ‘Nitrating Mixture’.]
benzene nitrobenzene
At a higher temperature, another H-atom can be replaced by NO2 group to produce dinitrobenzene.
nitrobenzene m-dinitrobenzene
Sulphonation
When benzene is heated with concentrated sulphuric acid at 353 K for 8 hours, benzene sulphonic acid is formed.
benzene benzene sulphonic acid
Oleum gives m-disulphonic acid.
benzene oleum benzene disulphonic acid
Mechanism
The accepted mechanism for sulphonation is given below:
Halogenation
In halogenations, the nature of the products depends upon the reaction conditions.
Benzene in the presence of a halogen carrier e.g., FeCl3 or iodine, reacts with chlorine and bromine even in dark and at room temperature to give mono- and di-halo derivatives,
benzene chlorobenzene
chlorobenzene o-dichlorobenzene p-dichlorobenzene
Bromine reacts with benzene to form bromo derivatives. Iodine does not react with benzene.
Mechanism
The accepted mechanism of substitutional halogenation of aromatic compounds is described below.
Friedel-Crafts reaction
This reaction is used for introducing an alkyl (R-) or acyl (RCO-) group into the benzene ring. Benzene, in the presence of anhydrous aluminium chloride (AlCl3), reacts with substances such as haloalkane (RX), alcohol (ROH) and acid chlorides (RCOCl) etc., to give the corresponding derivative of benzene. Some examples of alkylation are,
benzene chloromethane toluene
bromoethane ethylbenzene
These two reactions are called alkylation of benzene.
Some examples of acylation are,
acetophenone
Introduction of COCH3 group in benzene ring is called acylation of benzene.
Directive influence in arenes
During the formation of monosubstituted products in benzene as all the six hydrogens are equivalent any of the six positions can be occupied. But, when the monosubstituted product is to be converted into disubstituted one, the existing substituent present in the ring directs the incoming group to a particular position. This is referred to as directive influence of the group. Depending upon their directive influence, various groups/substituents/functional groups can be divided into two categories as described:
- The substituents or groups, which direct the incoming group to ortho and para positions are called ortho and para directing groups. For example:
-CH3,- C2H5-, -Cl, -OH, -Br, -NH2, -NHR, -NR2, -NHCOCH3, -OCH3 etc.
If we carry out nitration of toluene, the mixture of ortho and para nitrotoluenes are formed.
Toluene o-Nitrotoluene p-Nitrotoluene
These groups (except halogens) increase the electron density at the ring through resonance effect. Thus the reactivity of benzene ring towards electrophilic substitution reactions increases.
- The substituents or groups which direct the incoming group to meta position are called meta directing groups. For example:
-NO2, -CN, -CHO, -COR, -COOH, -COOR, -SO3H, etc.
The nitration of benzoic acid produces m-nitrobenzene.
Benzoic acid m-Nitrobenzoic acid
These groups withdraw the electrons from benzene ring through resonance effect, reducing the electron density at the benzene ring. They decrease the reactivity of benzene ring towards electrophilic substitution reaction and make it less susceptible to the electrophilic attack.
Oxidation
Benzene is slowly oxidised by chromic acid, acidified KMnO4 etc., to CO2 and H2O.
C6H6 + 15 [O]6CO2 + 3H2O DH = -650kJ
When benzene vapours mixed with air are passed over V2O5 (vanadium pentoxide) at 400°C, maleic anhydride is obtained.
maleic anhydride
Oxidation of alkyl sidechain
The alkyl sidechain in the molecule of an arene can be oxidised under different conditions.
- With hot acidified KMnO4 or K2Cr2O7, the sidechain gets oxidised to COOH group irrespective of the length of the sidechain. For example,
toluene benzoic acid
phenylethane benzoic acid
- With weak oxidising agents such as acidic manganese dioxide (MnO2) or chromylchloride (CrO2Cl2), the side chain is oxidised to aldehyde (-CHO) group.
Combustion of benzene and uses
Combustion of benzene
Benzene and its homologues are highly inflammable liquids and burn with a sooty flame.
2C6H6 + 15O2 12CO2 + 6H2O
Uses of benzene
- As an industrial solvent for fats and oils, rubber, resins etc.
- As a starting material for dyes, drugs, perfumes and explosives and polymers
- For dry-cleaning of woollen clothes.
- As a motor fuel along with petrol, under the name benzol.
Revision exercises
- How would you prepare ethyne in the laboratory?
- How would you obtain ethanol from ethyne? Give trhe equation of reaction.
- How would you distinguish ethyne from ethane?
- Describe Benzene and its properties.
- State five compounds of Benzene with their structure.
WEEK 10
INTRODUCTION
ALKANOLS
Classification, nomenclature and isomerism of alcohols
Introduction of alcohols
The hydroxy derivatives of aliphatic hydrocarbons (compounds having their carbon atoms in chains and not in the form of rings) are called alcohols. When one, two or more hydrogen atoms of a hydrocarbon are replaced by a corresponding number of hydroxyl groups (-OH), alcohols can be obtained.
Classification of alcohols
They can be classified as:
- Alcohols with one hydroxyl group – Monohydric alcohol
- Alcohols with two hydroxyl groups – Dihydric alcohol
- Alcohols with three hydroxyl groups – Trihydric alcohols
- Alcohols with four or more hydroxyl groups – Polyhydric alcohols
The saturated monohydric alcohols have the general formula CnH2n+1OH or ROH. Their functional group is -OH. They can be further classified into:
Primary Alcohol
Here the carbon atom bearing the hydroxyl group is attached to just one other carbon atom.
Secondary Alcohol
Here the carbon atom bearing the hydroxyl group is attached to two other carbon atoms.
Tertiary Alcohol
Here the carbon atom bearing the hydroxyl group is attached to three other carbon atoms.
Nomenclature of alcohols
In the common system, alcohols are named as alkyl alcohols. The word alcohol is added after the name of the alkyl group to which the hydroxyl group is attached. For e.g., CH3OH is methyl alcohol.
In the IUPAC system, the names of saturated alcohols are derived from corresponding alkenes by replacing ‘e’ of alkenes by ‘ol’
Some examples are shown below.
The numbering is done such that the carbon atom attached to the
-OH group gets the lowest number.
For naming polyhydric alcohols, the name of the alkane is retained and the ending -e is not dropped. Thus dihydric alcohols are named as alkane diols and trihydric alcohols are named as alkene triols.
The position of carbon atoms carrying -OH groups are indicated by locants written after the name of alkene. The number of hydroxyl groups is indicated by adding the multiplicative prefix di, tri, tetra etc., before the suffix-ol.
Isomerism of alcohols
Alcohols exhibit following types of isomerism:
- Chain isomerism
Alcohols with four or more carbon atoms exhibit this type of isomerism in which the carbon skeleton is different.
- Position isomerism
Alcohols with three or more carbon atoms can exhibit position isomerism. In this type of isomerism the position of the functional group i.e., the -OH group varies. In other words the carbon atoms to which the -OH group is attached is different.
- Functional isomerism
Alcohols with two or more carbon atoms can exhibit functional isomerism with ethers. Thus ethers and alcohols have the same molecular formula but have different functional groups, hence they are called functional isomers.
- Optical isomerism
Alcohols containing chiral centrescen exhibit enantiomerismor optical isomerism. The optical isomers can rotate the plane of plane polarized angles in different directions.
- represents an asymmetric carbon atom.
Preparation of monohydric alcohols
- From aldehyde and ketones
Aldehydes and ketones are reduced to the corresponding alcohols by
- a) Addition of hydrogen in the presence of catalysts like finely divided platinum, palladium, nickel and ruthenium.
This method is called catalytic hydrogenation.
- b) Treatment with chemical reagents such as sodium borohydride (NaBH4) or Lithium aluminium hydride (LiAlH4).
Aldehydes yield primary alcohols while ketones give secondary alcohols.
- From carboxylic acids and esters
Carboxylic acids are reduced to primary alcohols in the presence of strong reducing agent like lithium aluminium hydride.
The yield of alcohol here is high but LiAlH4 being an expensive reagent, this method is not commonly used.
Commercially acids are reduced to alcohols by converting them to the esters followed by their reduction using either:
- a) Hydrogen in the presence of a catalyst (Catalytic hydrogenation) or
- b) Sodium and alcohol.
From alkenes
- a) Hydration
Alkenes undergo hydration (addition of water across C=C bond) in the presence of dilute H2SO4 to produce alcohols. The alkyl hydrogen sulphate is formed which on hydrolysis with hot water gives alcohol.
The addition of water to the double bond is in accordance with Markownikov’s rule. The alkene is obtained by cracking of hydrocarbons. The alkene is then absorbed by passing it into sulphuric acid at 353 K and 30 atmosphere pressure. The acid is diluted and treated with stream to release the alcohol.
The preparation of ethyl alcohol is done starting with ethane.
Similarly isopropyl alcohol is prepared from Propene.
- b) Oxymercuration – demercuration
Alkenes react with mercuric acetate in presence of water to yield hydroxy mercurial compounds. These are reduced to alcohols by sodium borohydride.
This reaction gives a good yield of alcohol.
The alcohol obtained corresponds to Markownikov’s addition of water to an alkene.
- c) Hydroboration
Alkenes react with diborane (B2H6), which is an electron deficient molecule to yield alkylboranes (R3B). These are oxidised to alcohols on reaction with hydrogen peroxide in presence of alkali.
In each addition step, the boron atom is attached to the sp2 carbon atom that is bonded to greater number of hydrogen atoms. The hydrogen atom of the boron atom attaches to the other carbon of the double bond. Thus this is anti-Markovnikov’s addition.
During the oxidation of trialkyl borane, boron is replaced by -OH group.
The yield of alcohol in this method is good and the product is easy to isolate.
- d) From Grignard’s reagent
Grignard reagents (R MgX) are alkyl or aryl magnesium halides.
The C Mg bondin Grignard reagent is a highly polar bond as carbon is electronegative relative to electropositive magnesium. Due to this polar nature of C-Mg bond, Grignard reagents are very versatile magnets in organic synthesis.
Grignard reagents regents react with aldehydes and ketones to form products, which decompose with dil HCl or dil H2SO4 to give primary secondary and tertiary alcohols.
The overall result is to bind the alkyl group of Grignard reagent to carbon of the carbonyl group and hydrogen to oxygen. Formaldehyde gives primary alcohol where as all other aldehydes give secondary alcohols and ketones furnish tertiary alcohols.
Industrial preparation of ethanol
Ethanol can be manufactured by the fermentation of:
- Molasses
- Starch.
Slow decomposition of organic compounds is called fermentation. This is the principle behind souring of milk, batter, putrefaction of meat, and preparation of wine and vinegar. Fermentation was the earliest method used for preparing alcohol in industries. This is still used for the manufacture of alcohol and alcoholic drinks like beer, wine, brandy, etc,.
Raw materials
Cheap starchy materials like potatoes, maize, barley, rice etc.
OR Molasses, a byproduct of sugar industry.
From Molasses
The syrup left after the separation of cane sugar or beet sugar crystals from the concentrated sugar cane juice is called molasses. It is a dark coloured syrupy mass and contains about 30% of uncrystallizable sucrose and about 32% of invert sugar (a mixture of glucose and fructose). The different steps in the manufacture of ethanol by fermentation of molasses are:
Dilution
The molasses is diluted with water until a concentration of 8-10% sugar is obtained in solution. To discourage bacterial growth, this is acidified with a little sulphuric acid. If sufficient yeast (food for the ferment) is not present, a nutritive solution of ammonium salts is added.
Alcoholic Fermentation
Highly Magnified Yeast Cells
The dilute solution obtained as above is taken in big fermentation tanks and some yeast is added (5% by volume). The temperature is maintained at 330K and the mixture is allowed to stand for a few days. Fermentation sets in and the enzyme (organic catalyst) invertase present in yeast, converts sucrose into glucose and fructose. Zymase, another enzyme present in yeast converts glucose and fructose into ethanol and carbon dioxide.
The carbon dioxide formed is allowed to escape but air is not allowed to enter. In presence of air ethanol formed would be oxidised to acetic acid.
The fermentation is complete in 3 days. The carbon dioxide obtained as byproduct is recovered and can be sold.
Distillation
The fermented liquor contains 9-10% of ethanol and is called wash or wort. It is distilled in a Coffey still (Distillation of wash in a Coffey still) to remove water and other impurities present in wash. The Coffey still consists of two tall fractionating columns with perforated plates. These columns are called the analyser and the rectifier. This works on the counter-current principle as the steam and alcohol travel in opposite directions through the still.
Steam passes up the analyzer and takes away the alcohol vapors from the dilute alcohol that is coming down. The mixture leaves the analyzer at the top. It then enters the rectifier at the base. The mixture heats the wort flowing through the pipes on its way to the analyzer. The steam condenses and the alcohol vapors escaping near the top are condensed in the condenser. The distillate contains about90% alcohol and the residue left in the still is used as cattle feed.
Rectification
The alcohol obtained contains other impurities besides water. These impurities are further removed by fractional distillation. Low boiling impurities like acetaldehyde distil over as first fraction. The middle fraction contains about 93-95% alcohol and is called rectified spirit. Often, distillation and rectification is carried out in the same operation.
From Starch
Starchy raw materials
Wheat, barley, rice, maize and potatoes.
Conversion of starch into maltose
Conversion of starch into maltose or saccharification is carried out as follows:
Malting
Moist barley is allowed to germinate in dark at 290K. Germinated barley is called Malt and this is heated to 330K (to stop further germination). It is then crushed and extracted with water. This Malt extractcontains the enzyme diastase.
Mashing
To break the cell walls, starch is reacted with superheated steam. This exposes the starch inside that forms a paste like mass called Mash.
Hydrolysis
Mash and malt extract are treated together at 320-330K. In about half an hour, hydrolysis is complete and maltose is formed.
Alcoholic Fermentation
Maltose obtained from starch is fermented in the presence of yeast. Maltase present in yeast converts maltose into glucose. Another enzyme zymase present in yeast, then converts glucose into ethanol and carbon dioxide.
Subsequent distillation and rectification yields rectified spirit.
Physical properties monohydric alcohols
Alcohols and Phenols consist of two parts, an alkyl/aryl group and a hydroxyl group. The properties of alcohols and phenols are due to the -OH group.
The alkyl and aryl groups modify these properties.
- The lower members of alcohols are colourless, volatile liquids with a characteristic alcoholic smell and burning taste whereas higher alcohols are odourless and tasteless.
Higher alcohols having 12 or more carbon atoms are colourless waxy solids. Phenols are colourless, crystalline solids or liquids. (They may become coloured due to slow oxidation with air).
- Solubility of alcohols
The first three members are completely miscible with water. The solubility rapidly decreases with increase in molecular mass. The higher members are almost insoluble in water but are soluble in organic solvents like benzene, ether etc.
The solubility of lower alcohols is due to the existence of hydrogen bonds between water and polar -OH group of alcohol molecules. Phenols too are sparingly soluble in water.
The -OH group in alcohols and phenols contain a hydrogen bonded to an electronegative oxygen atom. Thus they form hydrogen bonds with water molecules.
The solubility of alcohols in water decreases with increase in molecular mass because the increase in molecular mass, the non polar alkyl group becomes predominant and masks the effect of polar -OH group.
In addition, among the isomeric alcohols, the solubility increases with branching of chain. As the surface area of the non-polar part in the molecule decreases, the solubility increases.
Phenols are sparingly soluble in water but readily soluble in organic solvents such as alcohol and ether.
Chemical properties of monohydric alcohols
In alcohols and phenols, -OH group is the functional group. Thus the chemical properties of alcohols generally involve the reactions of -OH group. They can undergo substitution as well as elimination reaction.
Reactions of alcohols are classified into three types.
1) Reactions involving cleavage of -OH bond.
Reaction with metals
Alcohols and phenols react with metals such as sodium, potassium and aluminium to yield corresponding alkoxides and hydrogen.
In addition to this, phenols react with aqueous sodium hydroxide to form sodium phenoxides.
The above reactions show that alcohols and phenols are acidic in nature. In fact, alcohols and phenols are Bronsted acids i.e., they can donate a proton to a strong base (B:).
On treating in alkoxide with water, the starting alcohol is obtained.
This reaction shows that water is a better proton donar than alcohol i.e., alcohols are very weak acids even feeble than water. They do not turn blue litmus to red but treated with active metals liberate hydrogen.
The acidic character of alcohols is due to the presence of polar O-H group. Due to greater electro negativity of oxygen atom, the shared pair between O and H is drawn towards the oxygen atom helping in release of H+ ion.
An electron – releasing alkyl group (-CH3, -C2H5) increases electron density over the oxygen atom tending to decrease the polarity of O-H bond. This decreases the acid strength. In addition, the alkoxide ion formed is also destabilised due to concentration of negative charge on oxygen atom by electron releasing inductive effect of alkyl group. Thus the release of H+ ion is difficult.
The acid strength of alcohols decreases in the following order:
The tertiary alcohols are least acidic while primary alcohols (with only one alkyl group) are most acidic.
Consider the reaction
This reaction shows that alkoxide ion is a better proton acceptor then hydroxide ion, which show that alkoxides are stronger bases (sodium ethoxide is a stronger base than sodium hydroxide)
The basic strength of the alkoxides follow the order
Alcohols act as Bronsted bases as well. It is due to the presence of unshared electron pairs over oxygen, which makes alcohols proton acceptor
Preparation and uses of ethan- 1, 2. diol (glycol)
A dihydric alcohol, ethanediol is prepared commercially by acid hydrolysis of epoxy ethane (ethylene oxide).
1,2 Ethanediol is a colourless syrupy liquid with a high boiling point of 470 K. Its high boiling point is due to the presence of intermolecular hydrogen bonding. It shows all the reaction of -OH group.
Ethenediol is used as a coolant in automobiles. It is used for making dacron a fibre used in making clothes.
- Alkanols belong to a group of organic (carbon) compounds known as alcohols.
- Alcohols contain the OH, hydroxyl (or hydroxy)1, functional group.
- Alkanols can be classified as primary (1o), secondary (2o) or tertiary (3o) depending on the location of the OH (hydroxyl or hydroxy) functional group.
Physical Properties of Alkanols:
- Boiling point of primary alkanols increases with increasing length of carbon chain (or molecular mass).
Boiling point of a primary alkanol is higher than the boiling point of the corresponding alkane.
- Simple alkanols are soluble in polar solvents like water.
Solubility of an alkanol in water decreases with increasing length of carbon chain.
Chemical Properties of Alkanols:
- Alkanols undergo complete combustion in excess oxygen to produce carbon dioxide and water.
Energy is also produced during the combustion of alkanols, making alkanols useful as fuels.
- Alkanols react with active metals to produce a salt (called an alkanolate2) and hydrogen gas.
- Alkanols react with alkanoic acids to produce esters.
- In the presence of a strong acid such as sulfuric acid, alkanols undergo elimination reactions to produce alkenes (dehydration of alkanols).
- Primary and secondary alkanols can be oxidised. Tertiary alkanols cannot be oxidised.
Classification of Alkanols
Alkanols can be classified as primary, secondary or tertiary depending on the location of the hydroxyl (OH) functional group.
Chemists use o notation to refer to primary, secondary and tertiary alkanols:
- primary alkanol ≡ 1o alkanol
- secondary alkanol ≡ 2o alkanol
- tertiary alkanol ≡ 3o alkanol
Classification (o) General Formula Location of -OH group
Primary 1o H
|
R- C -OH
|
H
-OH on a terminal (end) carbon atom
Secondary 2o H
|
R- C -OH
|
R’
-OH on a carbon atom is bonded to 2 other carbon atoms
Tertiary 3o R”
|
R- C -OH
|
R’
-OH on a carbon atom is bonded to 3 other carbon atoms
Examples:
Classification (o) General Formula Examples
Primary 1o H
|
R- C -OH
|
H
H
|
CH3-CH2-CH2- C -OH
|
H
butan-1-ol
(or 1-butanol) H
|
CH3-CH2-CH2-CH2- C -OH
|
H
pentan-1-ol
(or 1-pentanol)
Secondary 2o H
|
R- C -OH
|
R’
H
|
CH3-CH2- C -OH
|
CH3
butan-2-ol
(or 2-butanol) HO
|
CH3-CH2- C -CH2-CH3
|
H
pentan-3-ol
(or 3-pentanol)
Tertiary 3o R”
|
R- C -OH
|
R’
CH3
|
CH3- C -OH
|
CH3
2-methylpropan-2-ol
(or 2-methyl-2-propanol) OH
|
CH3-CH2- C -CH2-CH3
|
CH3
3-methylpentan-3-ol
(or 3-methyl-3-pentanol)
Physical Properties
Boiling Point
Alkanols are polar molecules: R- Oδ–Hδ+
where the carbon chain is represented by R and δ- represents a partial negative charge on the oxygen atom and δ+ represents a partial positive charge on the hydrogen atom attached to the oxygen atom of the alkanol.
Hydrogen bonding can therefore occur between the alkanol molecules:
R- Oδ- – Hδ+ where – represents a covalent bond between atoms within the molecule
and .. represents the hydrogen bond between molecules
In order to boil an alkanol, enough energy must be supplied to break both
a.the stronger hydrogen bonds between the polar parts of the molecules and
b.the weaker intermolecular forces (london or dispersion forces) between the non-polar carbon (alkyl) chains (R).
.
.
.
.
.
.
Hδ+ – Oδ- -R
Primary Alkanols
Preferred IUPAC name
(alternative IUPAC name) Functional name Formula Boiling
Point
(oC) Trend
methanol methyl alcohol CH3-OH 65 lowest As the carbon chain gets longer (molecular mass increases) the boiling point increases.
As the non-polar carbon chain length increases, the weak intermolecular forces (dispersion or london forces) holding the chains together weakly becomes increasingly significant so more energy is required to separate the molecules.
ethanol ethyl alcohol CH3-CH2-OH 78 ↓
propan-1-ol
(1-propanol) n-propyl alcohol CH3-CH2-CH2-OH 97 ↓
butan-1-ol
(1-butanol) n-butyl alcohol CH3-CH2-CH2-CH2-OH 117 ↓
pentan-1-ol
(1-pentanol) n-pentyl alcohol CH3-CH2-CH2-CH2-CH2-OH 138 ↓
hexan-1-ol
(1-hexanol) n-hexyl alcohol CH3-CH2-CH2-CH2-CH2-CH2-OH 157 highest
number of
carbon atoms
in carbon chain boiling point (oC)
alkane alkan-1-ol
1 meth -162 65
2 eth -88.6 78
3 prop -42.1 97
4 but -0.5 117
5 pent 36.1 138
6 hex 68.7 157
The boiling point of an alkanol is higher than the boiling point of the corresponding alkane because the energy required to break the hydrogen bonds between alkanol molecules is greater than the energy required to break the weak intermolecular forces between alkane molecules.
Members should log-in to use the graph.
Increasing the number of polar OH (hydroxyl or hydroxy) functional groups on alkanol molecules increases the boiling point of the alkanol as shown in the table below:
name formula boiling
point (oC) name formula boiling
point (oC)
ethanol
(ethyl alcohol) CH3-CH2-OH 78 propan-1-ol
(1-propanol) CH3-CH2-CH2-OH 97
ethane-1,2-diol
(1,2-ethanediol)
(ethylene glycol) HO-CH2-CH2-OH 197 propane-1,2,3-triol
(1,2,3-propanetriol)
(glycerol) H
| H
| H
|
HO- C – C – C -OH
|
H |
OH |
H
290
Ethane-1,2-diol (1,2-ethanediol or ethylene glycol) has more OH (hydroxyl or hydroxy) functional groups than ethanol.
More OH functional groups means that more hydrogen bonds can form between the molecules.
Since hydrogen bonds are a stronger intermolecular force than the dispersion (london) forces that act between the non-polar alkyl chains, more energy will be required to separate molecules of ethane-1,2-diol than needed to separate molecules of ethanol.
Ethane-1,2-diol has a higher boiling point that ethanol.
Similarly, propane-1,2,3-triol (1,2,3-propanetriol or glycerol) has 3 OH functional groups while propan-1-ol (1-propanol) has only 1 OH functional group.
More OH functional groups means that more hydrogen bonds can form between molecules of propane-1,2,3-triol than can form between molecules of propan-1-ol.
More energy will be required to separate molecules of propane-1,2,3-triol.
Propane-1,2,3-triol has a higher boiling point than propan-1-ol.
Solubility
For short chain alkanols, the ability of these alkanols to form hydrogen bonds with the polar water molecules is responsible for them being soluble in water (miscible with water).
R – Oδ- – Hδ+
.
.
.
H – Oδ- – Hδ+
Preferred IUPAC name
(alternative IUPAC name) formula Solubility
(g/100g water) Trend
methanol CH3-OH miscible
ethanol CH3-CH2-OH miscible
propan-1-ol
(1-propanol) CH3-CH2-CH2-OH miscible
butan-1-ol
(1-butanol) CH3-CH2-CH2-CH2-OH 8 more soluble
pentan-1-ol
(1-pentanol) CH3-CH2-CH2-CH2-CH2-OH 2.3 ↓
hexan-1-ol
(1-hexanol) CH3-CH2-CH2-CH2-CH2-CH2-OH 0.6 less soluble
As the number of carbon atoms in the carbon (alkyl) chain increases however, the weak intermolecular forces (London or Dispersion forces) acting between the non-polar alkyl chains become increasing important.
Long non-polar alkyl chains are more attracted to each other than they are to the polar water molecules, so that the solubility of the alkanol molecule in water decreases.
Chemical Properties
Combustion
Energy is released during the combustion of alkanols.
alkanol + excess oxygen gas → carbon dioxide + water + energy
no.carbon
atoms in
the chain primary
alkanol molecular
mass energy released
(kJ mol-1)
1 methanol
CH3OH 32 726
2 ethanol
CH3CH2OH 46 1367
3 propan-1-ol
CH3CH2CH2OH 60 2021
4 butan-1-ol
CH3CH2CH2CH2OH 74 2671
5 pentan-1-ol
CH3CH2CH2CH2CH2OH 88 3331
Increasing the length of the carbon chain attached to
the hydroxyl (OH) functional group increases the amount of
energy released when the primary alkanol combusts.
CnH2n+1OH + 3n/2O2(g) → nCO2(g) + (n+1)H2O + energy
CH3-OH + 3/2O2(g) → CO2(g) + 2H2O + 726 kJ/mol
C2H5-OH + 3O2(g) → 2CO2(g) + 3H2O + 1367 kJ/mol
C3H7-OH + 9/2O2(g) → 3CO2(g) + 4H2O + 2021 kJ/mol
C4H9-OH + 6O2(g) → 4CO2(g) + 5H2O + 2671 kJ/mol
Members should log-in to use the graph.
Incomplete combustion of an alkanol produces water and either carbon monoxide or solid carbon or both carbon monoxide and solid carbon.
Reaction with Active Metal
alkanol + active metal → salt + hydrogen gas
Active metals include Group 1 (IA or alkali) metals such as sodium and potassium.
The salt of an alkanol in IUPAC nomenclature is called a metal alkanolate (traditional name is a metal alkoxide).
The alkanolate ion has the general formula R-O-.
These metal alkanolate salts are named the same way as inorganic salts:
- the metal is named first
- followed by the name of the alkanolate ion.
The longer the carbon chain of the alkanol, the less vigorous the reaction between the alkanol and the active metal.
Sodium reacts readily with ethanol, but only sluggishly with butan-1-ol (1-butanol). alkanol alkanolate ion
(alkoxide ion)
methanol
CH3-OH methanolate
(methoxide)
CH3-O-
ethanol
CH3-CH2-OH ethanolate
(ethoxide)
CH3-CH2-O-
propan-1-ol
(1-propanol)
CH3-CH2-CH2-OH propan-1-olate
(propoxide)
CH3-CH2-CH2-O-
butan-1-ol
(1-butanol)
CH3-CH2-CH2-CH2-OH butan-1-olate
(butoxide)
CH3-CH2-CH2-CH2-O-
general word equation: alkanol + active metal → alkanolate + hydrogen gas
general chemical equation: R-OH + M → R-O-M+ + ½H2(g)
word equation example: ethanol + sodium → sodium ethanolate + hydrogen gas
chemical equation example: CH3CH2-OH + Na(s) → CH3CH2-O-Na+ + ½H2(g)
word equation example: ethanol + potassium → potassium ethanolate + hydrogen gas
chemical equation example: CH3CH2-OH + K(s) → CH3CH2-O-K+ + ½H2(g)
Reaction with Alkanoic Acids
Alkanols react with alkanoic acids to produce esters.
For this reason, the reaction between alkanols and alkanoic acids are usually referred to as esterification reactions.
general word equation: primary alkanol + alkanoic acid → ester + water
general chemical equation: R-OH + R’-COOH → R’COOR + H2O
word equation example: ethanol + propanoic acid → ethyl propanoate + water
chemical equation example: C2H5-OH + C2H5-COOH → C2H5-COOC2H5 + H2O
word equation example: propan-1-ol
(1-propanol) + acetic acid3
(ethanoic acid) → propyl acetate4
(propyl ethanoate) + water
chemical equation example: C3H7-OH + CH3-COOH → CH3-COOC3H7 + H2O
Dehydration of Alkanols
The dehydration of alkanols is an example of an elimination reaction. Water is eliminated during the reaction.
general word equation: alkanol hot conc sulfuric acid
———————> alkene + water
word equation example: ethanol hot conc sulfuric acid
———————> ethene + water
chemical equation example: CH3CH2OH hot conc H2SO4
———————> CH2=CH2 + H2O
word equation example: propan-1-ol
(1-propanol) hot conc sulfuric acid
———————> prop-1-ene
(propene) + water
chemical equation example: CH3CH2CH2OH hot conc H2SO4
———————> CH3CH=CH2 + H2O
Oxidation of Alkanols
Primary alkanols can be oxidised using a strong oxidising agent such as potassium permanganate solution or potassium dichromate solution..
Primary alkanols are first oxidised to the alkanal (aldehyde) which undergoes further oxidation to produce the alkanoic acid:
general word equation: 1o alkanol oxidising agent
——————> alkanal
(aldehyde) oxidising agent
——————-> alkanoic acid
(carboxylic acid)
word equation example: ethanol oxidising agent
——————> acetaldehyde5
(ethanal) oxidising agent
——————> acetic acid
(ethanoic acid)
chemical equation example: CH3CH2OH [O]
——————> CH2-HC=O [O]
——————> CH3-COOH
word equation example: propan-1-ol
(1-propanol) oxidising agent
——————> propanal oxidising agent
——————> propanoic acid
chemical equation example: CH3CH2CH2OH [O]
——————> CH3CH2-HC=O [O]
——————> CH3CH2-COOH
Secondary alkanols can be oxidised using a strong oxidising agent such as potassium permanganate solution or potassium dichromate solution.
The oxidation of a secondary alkanol produces an alkanone (ketone).
general word equation: 2o alkanol oxidising agent
——————> alkanone
(ketone)
word equation example: propan-2-ol
(2-propanol) oxidising agent
——————> acetone6
(propan-2-one)
chemical equation example: H
|
CH3- C -OH
|
CH3
[O]
——————>
CH3- C =O
|
CH3
word equation example: butan-2-ol
(2-butanol) oxidising agent
——————> butan-2-one
(butanone)
chemical equation example: H
|
CH3-CH2- C -OH
|
CH3
[O]
——————>
CH3-CH2- C =O
|
CH3
Tertiary alkanols cannot be oxidised using oxidising agents such as permanganate or dichromate
solutions.
REVISION EXERCISES
1.What is the general molecular formular of Alkanols?
2.Give the names and structural formulae for all the alkanols represented by the formula C3H8O.
3.Explain what happens when ethanol is refluxed with acidified sodium heptaoxodichromate(vi) solution for a long time.Give the equation of the reactions.
4.Give three industrial uses of alkanols.
Hope you got what you visited this page for? The above is the lesson note for Chemistry for SS2 class. However, you can download the free PDF file for record purposes.
If you have any questions as regards Chemistry lesson note For SS2 class, kindly send them to us via the comment section below and we shall respond accordingly as usual.